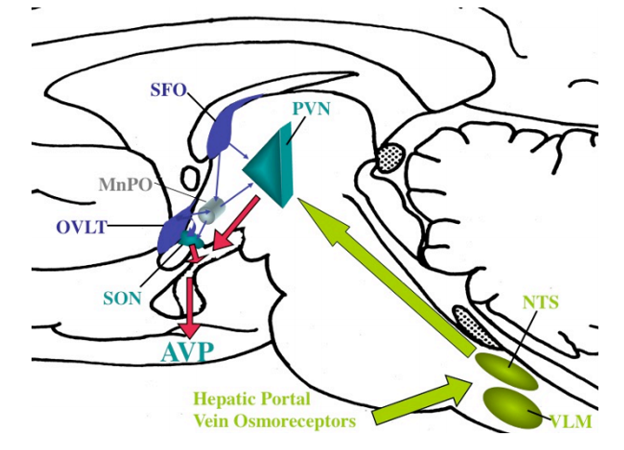
Glial-neuronal interactions in the median eminence and in contact with GnRH cell bodies may also be involved in regulating the delivery of GnRH to the portal system. In the median eminence, two mechanisms have been proposed (328-331). The first involves the release of glutamate, prostaglandins and growth factors from tanycytes that induce the secretion of GnRH from their axon terminals in the median eminence. The second involves plastic rearrangements between tanycyte end foot processes and GnRH axon terminals, allowing or disallowing secreted GnRH from entering portal capillaries. Thus, it is proposed during the preovulatory gonadotrophin surge, estrogen binds to alpha type estrogen receptors on tanycytes and induces tanycyte end feet retraction through PGE2-dependent production of TGFβ1, allowing GnRH axon terminals to establish better contact with the portal vessels (332). Estrogen may also affect the synthesis of adhesion molecules such as polysialylated neuronal cell adhesion molecule (PSA-N-CAM) and synaptic cell adhesion molecule (SynCAM) that facilitate glial-neuronal interactions and remodeling (328,329). The release of nitric oxide from portal vessel endothelial cells may also participate in tanycyte retraction by affecting actin cytoskeleton remodeling (332). Astrocytes have also been observed to enwrap GnRH perikarya and may similarly induce the release of GnRH through the release of PGE2 and/or TGFβ (331). It has also been hypothesized that astrocytes contribute to the mechanism whereby GnRH neurons are synchronized to generate the pulsatile release of the neuropeptide (331).
Mechanisms for reawakening of the reproductive axis during puberty is not fully understood, but appears to involve a decrease in transsynaptic inhibition to the GnRH neuronal system and increase in its stimulatory input from afferent neurons. GABAergic neurons are one of the major inhibitory inputs to the GnRH system and when inhibited, result in premature activation of the GnRH neuronal system. Increased stimulatory drive has been associated with glutamatergic transmission as well as increases in norepinephrine and NPY. Circulating levels of leptin also have an important role in the central modulation of puberty and reproductive function by food availability and nutritional status and can reverse the suppressive effects of undernutrition on the reproductive axis, but mediated primarily through kisspeptin neurons. Conversely, inhibition of reproductive function in association with stress appears to be mediated through CRH as suppression of gonadotropin secretion is reversible by administration of a CRH antagonist (333).
MODULATION OF PROLACTIN-REGULATING FACTORS
Prolactin secretion from the anterior pituitary is primarily under inhibitory regulation by dopamine neurons (A12 group of Dahlstrom and Fuxe) located in the arcuate nucleus. However, a number of prolactin-releasing factors have also been identified including TRH, oxytocin, VIP, vasopressin, histidine isoleucine, and serotonin, that can also trigger prolactin release under different physiologic circumstances following direct release into the portal system (334). For example, histidine isoleucine, which is co-expressed with CRH in parvocellular neurons in the PVN, and vasopressin are involved in stress-induced prolactin secretion through direct effects on lactotrophs. Serotonin has been implicated in lactation-induced prolactin secretion partly by stimulating TRH secretion from hypophysiotropic neurons, but also by inhibiting dopamine secretion and is further discussed below in the Lactation section.
Feedback control of prolactin secretion is mediated by a short-loop mechanism in which prolactin, itself, increases dopamine synthesis from the A12 neurons However, multiple neurotransmitter systems impinge on the A12 neurons that contribute to regulation of their neurosecretion including cholinergic neurons derived from the basal forebrain, hypothalamic glutamatergic and brainstem serotoninergic afferents that have activating effects and hypothalamic histaminergic and opiate peptide-containing afferents that have inhibitory effects (334).
Modulation of Vasopressin Secretion and Osmoregulation
Maintenance of the appropriate solute concentration in plasma (osmotic homeostasis) and plasma volume (volume homeostasis) is dependent upon two major factors, the perception of thirst and the ability to synthesize and secrete the antidiuretic hormone, arginine vasopressin from magnocellular neurons in the hypothalamic PVN (335). These two factors are closely interrelated such that amount of vasopressin circulating in the periphery is proportional to the plasma osmolality (336). Vasopressin induces cAMP and the translocation of specific aquaporin-2 water channels to the apical plasma membrane of tubular epithelial cells in the kidney, allowing water resorption (337,338). In addition, the rise in osmolality has independent behavioral effects. Thus, when plasma osmolality rises above basal levels, there is inducement to drink, shortly following the rise in vasopressin (335). While some vasopressin neurons in the PVN are intrinsically osmosensitive (339,340), the major mechanism of osmoregulation is via afferent pathways originating from osmoreceptor cells in other neuronal populations. These include inputs from the OVLT and the median preoptic nucleus, which if damaged, simultaneously abolish vasopressin secretion and thirst responses to hyperosmolality in both experimental animals and man (136,341). The SFO is also activated by a rise in osmolality and may contribute to vasopressin release through direct afferent projections to the PVN and/or to the OVLT using angiotensin II as a mediator (133-136,342). As mice with targeted disruption of the transient receptor potential (TRP) ion channels, TRPV1 and TRPV4, have impairment in vasopressin secretion and reduced drinking in response to hypertonic stimuli and show diminished cFos responses in the OVLT, these ion channels may be responsible for osmoreception (343). Along these lines, it particularly interesting that vasopressin can also be increased by hyperthermia (344,345), and that the trp genes are known to encode proteins involved in thermoregulation (see section E. Thermoregulation). Indeed, TRPV1 is required for thermosensory transduction of vasopressin secretion from isolated magnocellular neurons (346). Sodium channels may also contribute to the regulation of vasopressin secretion in response to hypernatremia by acting on neurons in the OVLT and SFO (347).
Whereas forebrain pathways communicate information about osmolality to the PVN, brainstem projections tend to carry nonosmotic, baroregulatory information, and important for vasopressin secretion, particularly in association with hypovolemia and hypotension (136,335). This information is carried through the vagus and glossopharyngeal nerves to the NTS and ventral lateral medulla, and then to the PVN through the ascending catecholaminergic pathways (Fig. 36). Magnocellular neurons in the PVN appear to be primarily innervated by the A1 catecholamine-producing cells in the ventral lateral medulla (247).