ABSTRACT
Endocrine hypertension belongs to the group of secondary forms of hypertension and mostly is caused by disorders of the adrenal gland. There are also nonadrenal endocrine conditions that can lead to hypertension secondary to hormonal imbalance and this chapter reviews how to best identify patients with and test for such disorders. For complete coverage of all related areas of Endocrinology, please see our FREE on line web-book, www.endotext.org.
INTRODUCTION
Hypertension (HTN) is a preventable contributor to disease and death in humans. HTN is commonly defined as a blood pressure (BP) ≥140/90 mm Hg for adults ≥18 years old based on the mean of ≥2 properly measured seated BP readings on each of 2 or more office visits. The overall prevalence of HTN among U.S. adults has not changed appreciably since 2009-2010, affecting ~29.1% of the population (1). The majority of patients with HTN (82.8%) are aware of their HTN and taking medication to lower it (75.7%), although BP control (<140/90 mm Hg) is suboptimal (51.9%) (1). Other studies have demonstrated BP control in < 1 in 3 patients (2, 3).
The prevalence of resistant HTN varies from 34-53% in different large studies: ALLHAT (34%), NHANES (53%) or Framingham Heart Study (48%) (4). Control to <140/90 mm Hg has been a matter of debate over the past decade. Recently, a large randomized trial of intensive versus standard BP control (SPRINT) among patients at high risk for cardiovascular events but without diabetes mellitus, showed that targeting a systolic BP <120 mm Hg, as compared with <140 mm Hg, resulted in lower rates of fatal and nonfatal major cardiovascular events and death from any cause (5). However, significantly higher rates of some adverse events, including hypotension and kidney injury were observed in the intensive-treatment group (5). In another study, the Heart Outcomes Prevention Evaluation (HOPE)-3 trial randomly assigned 12,705 participants at intermediate risk who did not have cardiovascular disease to rosuvastatin or placebo and to candesartan plus hydrochlorothiazide or placebo, and showed that there was a clear benefit of antihypertensive therapy in persons with a systolic BP of ≥140 mm Hg but no benefit on cardiovascular events (as a composite) in those with an initial systolic BP of <140 mm Hg (6). SPRINT and (HOPE)-3 have implications for antihypertensive therapy and the cutoff of BP target in general.
Table 1. Classification of Hypertension
|
||
Systolic (mm Hg) | Diastolic (mm Hg) | |
Normal | < 120 | < 80 |
Pre-hypertension | 120-139 | 80-89 |
Stage 1 hypertension | 140-159 | 90-99 |
Stage 2 hypertension | ≥ 160 | ≥ 100 |
The definition, diagnostic method and therapeutic targets for HTN differ between several recently published international and national guidelines (see Table 2).
Table 2. Selected Guidelines for the Diagnosis of Hypertension
|
|
Guidelines |
Definition, Diagnostic Method and Targets
|
2011 UK National Institute for Health and Clinical Excellence (NICE) (11)
|
• ≥ 140/90 mm Hg and ABPM (or home BP) >135/85 • Target: <140/90. ≥80 years old, <150/90
|
2013 European Society of Hypertension (12) |
• ≥140/90 on office ABPM (gold standard) • Ambulatory or home BPM for resistant HTN • Target: <140/90. ≥80 years old, SBP <140-150
|
2014 Joint National Committee (JNC 8) (9, 10) |
• Did not define HTN • Office ABPM favored • Ambulatory BPM is not mentioned • Target: <60 year, <140/90. ≥60 year, <150/90
|
2014 American Society of Hypertension - International Society of Hypertension (13) |
• ≥140/90 on office ABPM (gold standard) • Ambulatory or home BPM for resistant HTN • Target: <140/90. ≥80 years old, <150/90 |
2015 Canadian Hypertension Education Program (CHEP) (14)
|
• Daytime mean ≥135/85, 24 hour mean 130/80 on ABPM. Home BPM mean ≥135/85. Mean office BP >180/110. • Target: <140/90. ≥80 years old, SBP ≤150
|
Abbreviations: ABPM, ambulatory blood pressure measurement; HTN, hypertension; SBP, systolic blood pressure.
Adapted from Stergiou et al. (15)
Several new terms and definitions are proposed to further classify HTN, which has added more confusion to clinicians. Refractory HTN, difficult to control HTN, controlled or uncontrolled resistant HTN with ≥3, ≥4, or ≥5 drugs, pseudo-resistant HTN, and apparent and true resistant HTN (16), are among the many others used in the literature. Common terminologies used in clinical practice are:
- Resistant HTN: The diagnosis of resistant HTN should by definition rely on the inability of a well-constructed antihypertensive regimen to control BP (16). The American Heart Association defines resistant HTN as BP that remains above goal in spite of full doses of at least 3 antihypertensive medications, including a diuretic (17). This definition identifies patients who are at high risk of having reversible causes of HTN, such as primary aldosteronism. The definition also includes patients whose BP is controlled but require 4 or more medications to do so (“controlled resistant HTN”) and pseudo-resistant HTN. A lower cutoff for the diagnosis of resistant HTN (<130/80 mm Hg) could be used in patients with diabetes mellitus or kidney disease (i.e., with a creatinine level of >1.5 mg per deciliter [133 μmol per liter] or urinary protein excretion of >300 mg/24-hour period), despite adherence to treatment with full doses of at least 3 antihypertensive medications, including a diuretic (18).
- Pseudo-resistant HTN: This should defined as an 'apparent' lack of BP control in spite of full doses of at least 3 antihypertensive medications, including a diuretic.
- Masked HTN: This is defined as a normal BP in the office (<140/90 mm Hg), but an elevated BP out of the office (ambulatory daytime BP or home BP >135/85 mmHg) (19). Higher prevalence of masked HTN with poor cardiovascular score was found in both untreated subjects and treated hypertensives (20).
- White-coat HTN: This is defined as subjects with office BP ≥140/90 mm Hg and a 24-hour BP <130/80 mm Hg (21).
- Refractory HTN: This is defined as BP that remained uncontrolled in spite of maximal medical therapy after ≥3 visits to a hypertension clinic within a minimum 6-month follow-up period (22).
- Isolated systolic HTN: This is defined as a systolic BP >140 mm Hg and diastolic BP <90 mm Hg, which is predominantly present in elderly patients, although not uncommonly in young and middle-aged adults (23).
- Malignant/accelerated HTN: This is characterized by HTN with multi-organ involvement appearing over a short period of time, from a few weeks to a few months.
- Sustained HTN: This is defined as BP >150/100 mm Hg on each of three measurements obtained on different days without antihypertensive therapy, necessating screening for secondary causes, including primary aldosteronism (24).
Several factors may induce episodes of resistance to therapy in treated hypertensive patients. Common factors include poor adherence to drug therapy, periodic changes in dietary factors (e.g.: high sodium intake, high alcohol consumption, natural licorice [glycyrrhiza glabra]), rapid weight gain, periodic changes in concomitant drugs (nonsteroidal anti-inflammatory agents [NSAIDs], selective COX-2 inhibitors, sympathomimetic agents [decongestants, diet pills, and cocaine], stimulants), weight loss medications, herbal compounds (e.g.: arnica, bitter orange, ephedra, ginkgo, and ginseng), and secondary forms of HTN (16).
The causes of HTN are broadly divided into primary (essential, due to an unclear etiology) and secondary. Secondary forms refer to an underlying, potentially correctable diagnosis, and are listed in Table 3. Approximately 10-20% of adults with HTN have a secondary form, although there is compelling evidence that the figure is likely higher (25). The prevalence of secondary forms of HTN is dependent on age, co-existing comorbidities, such as atherosclerosis, and the criteria used for screening. One study found the prevalence of secondary HTN was 10.2%, divided into renovascular HTN (3.1%), primary aldosteronism (1.4%), Cushing syndrome (0.5%), pheochromocytoma (0.3%), primary hypothyroidism (3.0%) and chronic kidney disease (defined as a serum creatinine > 2.0 mg/dl) (1.8%) (26). The presence of atherosclerosis significantly increased the prevalence of renovascular HTN (9.5%) and chronic kidney disease (8.0%) (26).
Table 3. Common and rare causes of Endocrine Hypertension
|
|
Common causes
|
Rare causes |
· Renal vascular hypertension · Primary aldosteronism · Hypothyroidism · Thyrotoxicosis · Hypercalcemia · Pheochromocytoma and paraganglioma · Cushing Syndrome
|
· CAH: 11β-hydroxylase deficiency · CAH: 17α-hydroxylase deficiency · Familial hyperaldosteornism (e.g.: Type 1: Glucocorticoid-Remediable Aldosteronism) · Apparent mineralocorticoid excess · Liddle syndrome · Pseudohypaldosteronism Type 1 · Pseudohypaldosteronism Type 2 · Glucocorticoid Resistance syndrome · Growth hormone excess · Neuroendocrine tumors (“Carcinoid”) · Medullary thyroid cancer · Geller syndrome
|
Abbreviations: CAH, congenital adrenal hyperplasia.
CLINICAL FINDINGS AND OTHER CLUES TO IDENTIFY PATIENTS WITH ENDOCRINE HYPERTENSION
Several physical examination findings may point to the etiology for secondary forms of HTN. Table 4 provides a specific description of the clinical presentation of endocrine conditions related to HTN. Symptoms such as flushing and sweating may point toward pheochromocytoma and paraganglioma (27), while a renal bruit has been demonstrated in 87% of patients with renal artery stenosis (28). Laboratory abnormalities such as hypokalemia and metabolic alkalosis suggest primary aldosteronism due to increased renal hydrogen ion loss. Other clues that may point to the presence of endocrine forms of HTN are:
- The onset of HTN in young individuals (< 40 years) or after the age of 50 years
- Patients of African American descent that are at an increased risk for ARMC5 mutations leading to primary aldosteronism
- Worsening HTN despite maximum drug treatment (failing triple-drug therapy including a diuretic) or controlled BP (<140/90 mm Hg) on four or more antihypertensive drugs
- Sustained HTN, >150/100 mm Hg on each of three measurements obtained on different days
- HTN and spontaneous or diuretic-induced hypokalemia
- HTN and adrenal incidentaloma (bilateral or unilateral), defined as an asymptomatic adrenal mass detected on imaging not performed for suspected adrenal disease
- HTN and obstructive sleep apnea
- HTN and a family history of early-onset HTN or cerebrovascular accident at a young age (<40 years)
- First-degree relatives of patients with endocrine HTN
- Skin lesions (pheochromocytoma and paragangliomas [PPGLs], neurofibromatosis 1 [NF1], multiple endocrine neoplasia types 1 or 2 [MEN1, MEN2]): retinal angiomas, marfanoid body habitus, mucosal neuromas on eyelids/tongue, café-au-lait spots, axillary frecking, angiofibromas, or collagenomas
- Worsening glycemic control and/or spinal osteoporosis (Cushing syndrome)
- MEN2 with symptoms and signs of medullary thyroid carcinoma, PPGLs and/or primary hyperparathyroidism
- Hemangioblastomas (brain, spinal cord, retina), endolymphatic sac tumors, renal cancer, pancreatic/renal/male genital tract cysts in von Hippel-Lindau (VHL) syndrome associated with PPGLs
- Renal cancer associated with PPGLs in patients with germline mutations in SDHx
- Gastrointestinal stromal tumors and PPGLs
- Signs related to MEN1: primary hyperparathyroidism (hypercalcemia and/ or nephrolithiasis, osteoporosis), pituitary tumors (visual field defect, galactorrhea, impotence, headache) etc.
Table 4. Clinical Findings in Patients with Endocrine Hypertension
|
|
Condition |
Clinical presentation
|
Renal Vascular Hypertension |
Sudden increase in BP while controlled by medication, abdominal bruit (holosystolic, high-pitched) with radiation to the flanks
|
Primary Aldosteronism |
Sustained, resistant or mild hypertension, muscle weakness, hypokalemia, metabolic alkalosis
|
Thyrotoxicosis
|
Isolated systolic hypertension, tremors, palpitations, atrial fibrillation, thyroid bruit, Graves’ extrathyroidal manifestations (orbitopathy, dermopathy, acropachy)
|
Cushing syndrome | Fatigue, weight gain, round face, proximal myopathy, skin thinning, ecchymosis, hirsutism, fat pads (supraclavicular, dorsocervical, temporal fossae), plethora, purple or red striae (>1cm) |
Pheochromocytoma and Paraganglioma |
Headache, palpitations, sweating, pallor, paroxysmal, resistant, mild hypertension, hypotension
|
CAH: 11β-hydroxylase deficiency |
Classic form: virilization of the external genitalia in 46,XX newborn females, and precocious pseudopuberty in both sexes.
Nonclassic form: extremely rare, presents with hyperandrogenism during childhood
|
CAH: 17α-hydroxylase deficiency |
Classic presentation: phenotypic female (46,XX or 46,XY) with hypertension, hypokalemia and absence of secondary sexual characteristics.
Partial 17-OHD: 46,XY with undervirilization and ambiguous genitalia.
|
Familial Hyperaldosteronism E.g.: Type I: Glucocorticoid-Remediable Aldosteronism (GRA) |
Early onset of hypertension, presence of family history of mortality or morbidity from early hemorrhagic stroke
|
Liddle syndrome | Severe hypertension, hypokalemia, and metabolic alkalosis |
Apparent Mineralocorticoid Excess | Growth retardation, short stature, hypokalemia |
Pseudohypaldosteronism Type 2 | Short stature, hyperkalemic metabolic acidosis, normal aldosterone levels |
Glucocorticoid Resistance Syndrome
|
Ambiguous genitalia, precocious puberty, acne, hirsutism, oligo/anovulation, hypertension |
COMMON CAUSES OF ENDOCRINE HYPERTENSION
Renal Vascular Hypertension (RVH)
RVH is one of the commonest types of secondary HTN that can be correctable with specific therapy. Most cases of RVH are caused by atherosclerotic renal artery stenosis (ARAS), fibromuscular dysplasia (FMD), vasculitis, thromboembolism and aneurysms. The renal arterial lumen must be decreased ≥ 70% to cause or worsen HTN. Most patients with RVH or renal artery stenosis (RAS) are >50 years old and have ARAS, while younger patients usually have renal artery FMD. Prevalence of RAS is estimated to be between 2% in unselected hypertensives and 40% in older patients with other atherosclerotic comorbidities (26, 29, 30). RVH is probably the third most common correctable cause of secondary HTN after OSA and primary aldosteronism. Table 5 lists the clinical and biochemical findings that should alert the clinician to screen for RVH:
Table 5. Clinical and biochemical findings associated with an increased possibility of RVH (31-34) |
• Moderate - severe or refractory HTN in patients with: · Multidrug (>3 agents) antihypertensive therapy · Recurrent episodes of flash pulmonary edema · Evidence of generalized vascular disease (CAD) or diffuse atherosclerosis · Unilateral small kidney (≤9 cm), or a difference in renal size of more than 1.5 cm that have no other explanation • Age/sex (young women <30 years old, think FMD, older men, think ARAS) • Sudden onset of severe HTN (≥160/100 mm Hg) after the age of 55 years • Elevation of serum creatinine ≥30% after administration of ACEi or ARB • Malignant HTN • Abdominal bruit (86% of cases)
|
Abbreviations: ACEi, angiotensin-converting enzyme inhibitor; ARAS, atherosclerotic renal artery stenosis; ARB, angiotensin II receptor blocker; CAD, coronary artery disease; FMD, fibromuscular dysplasia; HTN, hypertension.
The suggested work-up for RVH/RAS greatly depends on the degree of clinical suspicion. In general, a low index of suspicion does not require work-up. However, it is important to note that RVH is underdiagnosed and may be asymptomatic for several years. The American College of Cardiology/American Heart Association Guidelines (35) for screening of RVH/RAS recommends an invasive work-up when a corrective procedure is intended to be employed for clinically significant RVH. Individuals with moderate suspicion of RVH/RAS should undergo screening and confirmatory testing (see Figure 1).
The gold standard test for screening of RVS is renal arteriography. Noninvasive tests for diagnosing of RVH with a good sensitivity and specificity are Gadolinium enhanced magnetic resonance angiography (MRA), computed tomography angiography (CTA) and duplex ultrasonography. In presence of a positive screening test, renal arteriogram would help confirm the diagnosis and localize the site of the stenotic renal blood vessel. A schematic screening/diagnostic approach based on the degree of probability of clinical suspicion is described in Figure 1.
Plasma renin activity (PRA) as a screening test in patients with suspected RVH is underappreciated. PRA are within reference range in ~50% of patients with RVH while, conversely, increased levels may be found in ≤10% of patients with primary HTN (36-38). RVH leads to a low-pressure state within the afferent renal arterioles at the site of renin secretion, resulting in a log-unit (>10-fold) increase in plasma renin. Although no studies to date have evaluated the overall sensitivity and specificity of plasma renin as a diagnostic test in patients with RVH, the recognition of a plasma renin that is many-fold higher than the normal range, after accounting for false positives such as antihypertensive therapy, may be useful, particularly if the diagnosis was not appreciated on imaging (37). The sensitivity and specificity (39-47) of various tests in the workup of RVH/RAS are outlined in Table 6.
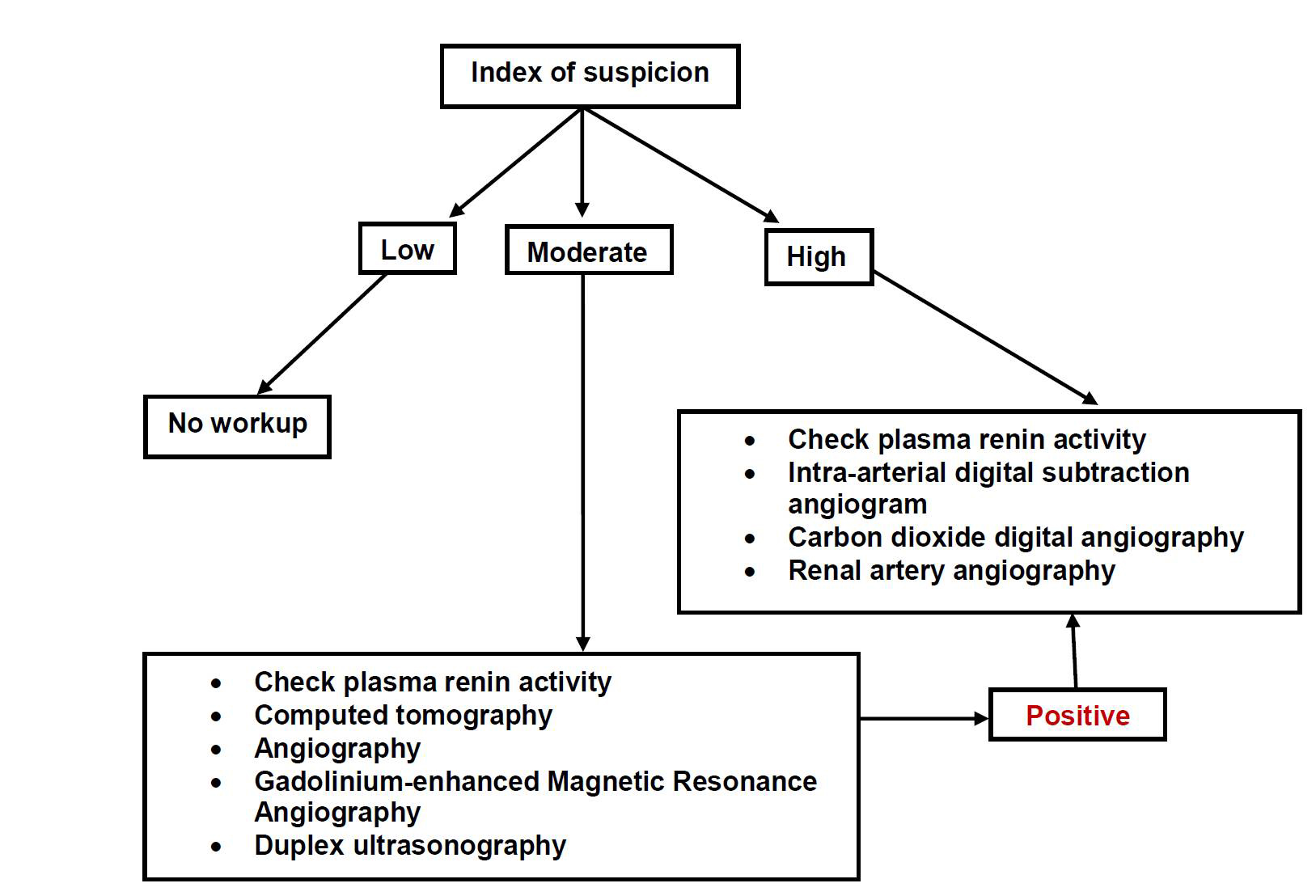
Figure 1. Flow chart for the diagnostic work-up of RVH.
Table 6. Tests used in the diagnosis of RVH | ||
Test | Sensitivity % | Specificity % |
Renal artery angiography | 100 | 100 |
Intra-arterial digital subtraction angiogram (DSA) | 94 | 97 |
Carbon dioxide digital angiography | 83 | 99 |
Computed tomography angiography (CTA) | 91 | 93 |
Gadolinium enhanced Magnetic resonance angiography (MRA) | 96-100 | 71-96 |
Duplex ultrasonography | 85 | 92 |
Captopril renography | 57-94 | 44-98 |
Captopril test | 15-68 | 76-93 |
The gold standard for confirming the diagnosis of RAS is a renal angiogram. Renal angiogram, intravenous subtraction angiography, intra-arterial digital subtraction angiography (DSA), or carbon dioxide angiography are imaging tests used in the diagnosis of RVH. Conventional aortography and intra-arterial digital subtraction angiogram (DSA) are considered the best tests offering high-quality radiographic images of the renal artery. Both, intra-arterial DSA (use less iodinated contrast ~25 mL) and carbon dioxide angiography are recommended in patients with deranged renal function. Intravenous DSA has a lower sensitivity and specificity compared with DSA and is falling out of favor. As an invasive procedure renal angiogram carry the risk of infections, cholesterol emboli and contrast-induced nephropathy.
Computed tomography angiography is a first line screening modality of RVH. Single breath-hold image detection and multidetector (MDCT) imaging have consistently improved the image acquisition and resolution with a better visualization of proximal/distal renal arteries. Spiral CT scan with angiography uses small amounts of IV iodinated contrast and is less invasive than arteriography. The use of iodinated compounds in subjects with poor renal function can result in contrast induced nephropathy. In patients with kidney failure the accuracy of this technique is impaired. Contrast allergies, anaphylaxis, and radiation exposure have to be considered in selected patients.
Gadolinium-enhanced MRA has one of the highest diagnostic performances for the detection of RAS among the non-invasive tests for RAS. The method is sensitive for imaging of proximal renal arteries but is suboptimal for significant distal lesions, accessory renal arteries and FMD (45). The use of 3-D gadolinium-enhanced MR (GEMR) imaging has improved the sensitivity and specificity of the method (>90%). Although MRA is considered as a first line investigation in patients with RVH, there are contraindications that limit its use in certain groups of patients: history of claustrophobia, metallic implant (pacemaker, surgical clips on vascular aneurysms), and pregnancy. The administration of gadolinium during MR imaging has caused nephrogenic systemic fibrosis in cases with acute/or chronic kidney disease and eGFR <30 mL/min (48, 49).
Duplex ultrasound is used as a screening test or for detection of recurrent stenosis of renal arteries in patients who underwent angioplasty/surgery. However, it is less sensitive in obese patients and the results are operator dependent (47). Using B-mode imaging/Doppler the operator can visualize main renal arteries and assess intrarenal pressures and velocities. Peak systolic velocity (>200 cm/sec.), renal-aortic ratio (≥3.5) and acceleration index (> .07 sec) are used to evaluate the presence of absence of a renal stenosis of >60% and end diastolic renal artery velocity of >150 cm/s for a stenosis of ≥80% (41, 44, 45).
Captopril renography is a noninvasive test to assess renal function. The administration of captopril orally (25-50 mg) 1 hour before the isotope injection increases the sensitivity of this test. Glomerular filtration can be estimated by measuring the excretion of Tc99m DTPA, Tc99m MAG3 or OIH (41, 46, 50). The test is considered positive (especially in unilateral RAS) when there is decreased relative uptake of the isotope with one kidney accounting for <40% of the total eGFR or a delayed peak uptake of the isotope of ≥10-11 minutes (50, 51). Limitation of the method include: creatinine ≥2 mg/dL and bilateral RAS.
Captopril test is used as a screening test. ~50% of patients with RVH have an increased PRA. PRA is measured before and 2 hours after oral administration of captopril 25 mg in seated position. Patients with RVH/RAS respond by increasing PRA >12 ng/ml/hr with an absolute increase >10 ng/ml/hr. Some antihypertensive therapy (ACEi, β-blockers, diuretics) should be stopped before testing. This method has a decreased sensitivity and specificity when compared with captopril renography, duplex ultrasound, CTA and MRA.
Cushing Syndrome
Endogenous Cushing syndrome (CS) is characterized by a constellation of signs and symptoms due to prolonged and high exposure of a variety of tissues to glucocorticoids. The incidence of endogenous CS is ~2-3 cases per 1 million inhabitants per year (52, 53). Endogenous CS is broadly divided into ACTH-dependent (~85% pituitary adenoma [Cushing disease], <5% ectopic ACTH secretion (54)) or ACTH-independent (~10-15% adrenal overproduction of glucocorticoids from an adrenocortical adenoma, hyperplasia or carcinoma). ACTH-independent causes of CS are classified on the basis of their radiographic and biochemical characteristics as being either functional or nonfunctional and benign or malignant. Approximately 75–90% of ACTH-independent causes of CS are due to unilateral and benign cortisol-producing adenomas, with the remaining majority due to bilateral adrenocortical hyperplasias (BAH) (55, 56).
BAH are divided into micronodular (<1 cm in diameter), macrocronodular (>1cm in diameter) or non-nodular. Briefly, the micronodular subtypes are usually diagnosed in children and young adults, and are either pigmented (primary pigmented nodular adrenocortical disease [c-PPNAD]) as seen in familial cases in the context of Carney complex, or isolated (i-PPNAD) when nonsyndromic, and not pigmented (iMAD; isolated massive adrenocortical disease). The macronodular subtypes, which are usually diagnosed in adults > 50 years old, may be sporadic or familial. Primary bilateral macronodular adrenocortical hyperplasia (PBMAH) was first described in 1964 (57), and was previously called massive macronodular adrenocortical disease (MMAD), bilateral macronodular adrenal hyperplasia (BMAH), or ACTH-independent macronodular adrenocortical hyperplasia (AIMAH) (58). PBMAH may be syndromic, as seen with mutations in ARMC5, APC, MEN1, FH and the Carney triad, Carney-Stratakis syndrome, and hereditary nonpolyposis colorectal cancer (55, 58-61). Other subtypes of macronodular PBMAH include primary bimorphic adrenocortical disease (PBAD), as seen in McCune-Albright syndrome, and lesions with G-protein-coupled receptors that produce excess cortisol only in response to certain endogenous factors (e.g.: gastrointestinal inhibitory polypeptide, GIP), as seen with food-dependent Cushing syndrome (FDCS).
CS is associated with HTN in ~80% of adult cases and ~50% of children (62-65); CS is more likely if the onset of HTN (among other signs or symptoms) is at a younger age. Several clinical features should be considered when evaluating individuals for the presence of this CS (62-64):
Patients with features very suggestive of hypercortisolism:
- Abnormal fat distribution, particularly in the supraclavicular, dorsocervical and temporal fossae
- Facial rounding with plethora
- Proximal myopathy
- Easy bruising
- Wide (>1 cm) purple striae
- Decreased growth rate with weight gain in children
- Menstrual irregularities
Patients with unusual features for their age group:
- HTN in young individuals or resistant to therapy
- Adrenal incidentalomas
- Metabolic syndrome X
- Hypogonadotropic hypogonadism
- Spontaneous fracture in a young individual
- Kidney stones
- Cyclicity in symptoms
- Hypokalemia
- Peripheral edema
- Psychiatric comorbidities
- Obesity
- Impaired short term memory
- Female balding
- Type 2 diabetes
- Unusual infections
Clinical characteristics of CS vary based on the duration and cyclicity of high cortisol level exposure. When cortisol levels are mildly/or intermittently increased (cyclical), clinicians face a diagnostic challenge. It is important to distinguish these symptoms/signs in order of their frequency: increased fatigue (sensitivity ~100%), generalized obesity (sensitivity ~51-90%), round face (sensitivity ~88-92%), plethora (sensitivity ~78-94%), HTN (sensitivity ~74-90%), weakness, especially of proximal muscles (sensitivity ~56-90%), thinness and fragility of skin (sensitivity ~84%), and hirsutism (sensitivity ~64-84%) (62, 65). Facial plethora is also one of the earliest described clinical features of CS (27, 66).
Common screening/diagnostic tests:
The initial screening test for CS should be based on the suitability for a given patient (see Figure 2). The tests recommended by the Clinical Practice Guidelines of the Endocrine Society (65) are: late-night salivary cortisol (LNSC, two measurements), 1-mg overnight dexamethasone suppression test (ODST), urine free cortisol (UFC; at least two measurements) and the longer low-dose DST (LLDST, 2 mg/d for 48 hours). A random serum cortisol or plasma ACTH levels, 8-mg DST, urinary 17-ketosteroids or the insulin tolerance test should not be used to screen for CS. Screening for aberrant expression of GPCRs in adrenocortical tumors and hyperplasia could be considered (67) in a select group of patients. The clinician should be aware of any current or recent use of oral, skin creams, rectal, inhaled, topical, herbal or injected glucocorticoids before biochemical testing to avoid false positives.
Assays differ widely in their accuracy, and should be chosen on the basis of their performance. Thus, knowledge of assay variability, functional limit of detection, precision and normal ranges should be carefully assessed to assist in the interpretation of the test results. Antibody-based immunoassays (RIA and ELISA) can cross-react with cortisol metabolites and synthetic glucocorticoids while structurally based assays (HPLC and LC-MS/MS) do not pose this problem and are the method of choice for detection of cortisol and/or other metabolites.
Late night salivary cortisol (LNSC) - Patient with CS have an impaired diurnal variation of cortisol. The loss of circadian rhythm with absence of a late-night cortisol nadir is a consistent biochemical abnormality in patients with CS (65, 68, 69). Since biologically active free cortisol in the blood is in equilibrium with cortisol in the saliva, then measurement of a late night salivary cortisol (LNSC) level by liquid chromatography–tandem mass spectrometry (LC-MS/MS) can be employed as a screening test for CS. 0.5 mL (minimum 0.2 mL) of saliva is necessary for the test. Basic instructions for collection includes: no food, smoking (ideally avoided on the day of testing), chewing tobacco/licorice (contains the 11β-hydroxysteroid dehydrogenase type 2 inhibitor glycyrrhizic acid) or fluids for 30 minutes to 1 hour prior to collection; avoid any activity that can cause gums to bleed, including brushing and flossing of teeth, or stress; the saliva should be collected 10 minutes after rinsing the mouth with water; the swab is placed under the tongue until well saturated approximately one minute; the specimen can be placed in room air for up to 5 days, and refrigerated for 7 days. Two saliva samples on two separate evenings between 2300 and 2400 hours should be collected because the hypercortisolism of CS can be variable, and this strategy increases confidence in the test results. Levels at midnight ≤0.09 mcg/dL (see questdiagnostics.com) are considered normal. The timing of the collection should be adjusted to the time of sleeping for shift workers or those with variable bedtimes. One study found that in men ≥60 years, 20% of all participants and 40% of diabetic hypertensive subjects had at least one elevated LNSC (70), which questions its utility as a screening test in this age group. LNSC is useful in detection early recurrence from CS in the postoperative period where urinary free cortisol and morning cortisol levels may be normal. If there is a normal diurnal rhythm (i.e. an appropriately low LNSC), then remission is likely (62, 64, 65). LNSC yields a 92–100% sensitivity and a 93–100% specificity for the diagnosis of CS (65).
1-mg Overnight Dexamethasone Suppression Test (ODST) - Patients with CS fail to suppress ACTH secretion from the pituitary gland when low doses of the synthetic glucocorticoid dexamethasone are given. This test entails administration of 1mg of dexamethasone at 2300 hours the night before a morning (0800 hours) blood sample for serum cortisol is drawn, simultaneously with a dexamethasone level (if feasible) to ensure adequate plasma concentrations [>5.6 nmol/L (0.22 μg/dL)] (71). Variable absorption and metabolism of dexamethasone may influence the result of both the 1-mg ODST and the longer low-dose DST (LDDST; 2 mg/d for 48 h). Patients should avoid eating or drinking for 12 hours before the morning blood test. Drugs such as phenytoin, phenobarbital, carbamazepine, rifampicin, and alcohol induce hepatic enzymatic clearance of dexamethasone, mediated through CYP3A4, thereby reducing the plasma dexamethasone concentrations leading to false positives (65). Dexamethasone clearance may be reduced in patients with liver and/or kidney failure. Interpretation of the serum cortisol has many caveats. The serum cortisol assay measures total cortisol, which is not an adequate representation of the biologically relevant free cortisol levels in conditions that cause cortisol binding globulin (CBG) deficiency (e.g.: nephrotic syndrome, cirrhosis, critical illness, postoperative period, CBG deficiency or malnourished states) or excess (e.g.: obesity, pregnancy, oral contraceptives, and estrogen therapy). False-positives for ODST are seen in 50% of women taking oral contraceptives, and should be withdrawn for 6 weeks before testing or retesting (72). Certain conditions associated with abnormal cortisol levels need to be excluded: alcoholism, major depression, stress, thyrotoxicosis, poorly controlled diabetes mellitus, pregnancy or kidney failure. Morning cortisol levels >1.8 mcg/dL (50 nmol/L) are considered positive (65). If an increased specificity (95-100%) is sought, the longer LDDST (2 mg/d for 48 h) could be employed (73), or a higher serum cortisol threshold for the 1mg ODST is used (74). This is particularly useful in the evaluation of adrenal incidentalomas where a cutoff of >5 mcg/dL (137.95 nmol/L) increases specificity for the detection of autonomous cortisol secretion (75). Fast-acetylators of dexamethasone may have a false positive test with the 1 mg ODST, which can be overcome with the longer LDDST. DST is not the screening test of choice in pregnancy, epilepsy, and cyclic CS. DST is the test of choice in renal failure and in the evaluation of an adrenal incidentaloma for autonomous cortisol secretion (so called mild or subclinical CS).
Urine Free Cortisol (UFC) - Unlike serum cortisol, UFC provides an integrated assessment of cortisol secretion that is not bound to CBG over a 24-hour period. Therefore, UFC is not affected by conditions and medications that alter CBG. Two UFC samples should be collected, with the first morning void discarded so that the collection begins with an empty bladder, up to and including the first morning void on the second day (65). Patients should not drink excessive amounts of fluid and to avoid the use of any glucocorticoid preparations. Because the hypercortisolism of CS can be variable, at least two collections should be performed, which increases confidence in the test results (65). Values above the upper limit of normal for the particular assay is considered positive, provided the creatinine shows that the collection is complete and that the urine volume is not excessive (>5L) (65). Pseudo-Cushing syndrome is associated with false positive UFC’s and should be considered on the differential. UFC appears to be less sensitive than the 1 mg DST or LNSC for the identification of autonomous cortisol secretion in the setting of an adrenal incidentaloma (65). Upper limits of normal are much lower with HPLC or LC-MS/MS than in antibody-based assays (as low as 40% of the value measured by RIA) (76).
Plasma ACTH - A serum ACTH level could help narrow the differential diagnosis of hypercortisolemia (ACTH-dependent vs. independent) after the diagnosis has been established. Immunochemiluminometric assays detect intact ACTH; 1.5 ml frozen EDTA plasma (0.3 ml minimum) is collected between 7:00-10:00 a.m., transferred on ice, and centrifuged immediately after collection to separate plasma from cells. The reference range for 3-17 years is 9-57 pg/mL, and ≥18 years is 6-50 pg/mL (see questdiagnostics.com). Elevated levels are seen in ectopic ACTH and Cushing’s disease, unless cyclicity is present, while suppressed levels are seen in ACTH-independent causes, such as CS due to adrenocortical tumors and hyperplasia. An ectopic ACTH-secreting pheochromocytoma from the adrenal glands is an exception to the rule. False-positives are not uncommon, and could be from errors in sample transfer and processing, assay interference (e.g.: 5 mg/day of biotin or presence of monoclonal mouse antibodies), and stress.
Corticotropin-releasing hormone (CRH) stimulation test - This test is useful for differentiating between ACTH-dependent from ACTH-independent CS. Human and ovine CRH are commercially available and are given intravenously (bolus) at a dose of 1 µg/kg body weight. ACTH and cortisol levels are measured before (-5, 0 minutes) and after (15, 30, 45, 60, 90, and 120 minutes) the administration of CRH. Some studies suggested that the measurements of ACTH and cortisol before and after 15, 30 minutes and 45, 60 minutes, respectively, are sufficient to diagnose patients with ACTH-dependent CS (77, 78). A rise in cortisol >20% and ACTH >35% in comparison with baseline levels is diagnostic for Cushing disease, with a sensitivity of 93% and a specificity of 100% (77).
Inferior petrosal sinus sampling (IPSS) - Inferior petrosal sinus ACTH sampling after CRH stimulation is the best method available for the intra-pituitary localization of microadenomas causing Cushing’s disease. This test also helps distinguish Cushing’s disease from ectopic ACTH secretion, provided that the appropriate technique of blood sampling is used meticulously (79). Bilateral IPSS and simultaneous peripheral ACTH sampling at baseline, 3 and 10 minutes after intravenous administration of ovine CRH (1 µg/kg) is performed, and baseline and/or stimulated IPS-to-peripheral ACTH ratios are calculated. A post-IPS-to-peripheral ACTH ratio >2 is sufficient for diagnosing Cushing’s disease (80). In about 70-80% of the cases, a ratio of greater than 1.4 between the right and left inferior petrosal sinuses confirms the presence of a microadenoma (79, 81, 82). Anomalous venous drainage, abnormal venous anatomy, and lack of expertise can lead to false-negative IPSS results and thereby disease misclassification. Prolactin measurement during IPSS can improve diagnostic accuracy and decrease false negative results (83).
Imaging modalities in Cushing syndrome:
Pituitary MRI pre- and post-gadolinium enhancement - MRI is the modality of choice in the evaluation of the pituitary gland and surrounding tissues. MRI provides excellent anatomical tissue discrimination without exposure to ionizing radiation. Sagittal and coronal planes are considered the most accurate in evaluating the anatomy of the pituitary gland and other CNS structures. When Cushing disease is suspected, contrast-enhanced magnetic resonance imaging (MRI) is recommended. T1-weighted (T1W) sequences and/or spoiled gradient recalled acquisition (SPGR) techniques provide the best images of the sella. 95% of microadenomas appear hypointense with no post-gadolinium enhancement in relation with normal surrounding tissues on T1W sequences (84-86). Only ~60-80% of pituitary adenomas are detected and ~10% of healthy individuals have abnormal findings (incidentalomas) on MRI (87). Diffuse hyperplasia of ACTH-producing cells and small microadenomas may not be seen on conventional or enhanced MRIs. Other techniques (IPSS or integrated 18F-FDG PET/CT) may be employed to increase the odds of disease detection. Dynamic MRI may further increase the detection rate of pituitary microadenomas at the expense of specificity.
High-resolution chest, neck, and/or abdominal CT - This technique may detect tumors in ectopic or adrenocortical areas. Small lesions (<1 cm) could be missed (bronchial carcinoids, pancreatic neuroendocrine tumors). The sensitivity is lower than MRI (~50%) (88).
Nuclear imaging - These techniques include 111In-pentetreotide (OCT), 131I/123I-metaiodobenzylguanidine, 18F-fluoro-2-deoxyglucose-positron emission tomography (FDG-PET), 18F-fluorodopa-PET (F-DOPA-PET), 68Ga-DOTATATE-PET/CT or 68Ga-DOTATOC-PET/CT scan (68Gallium-SSTR-PET/CT), which may be used in select cases, primarily for the detection of ectopic ACTH tumors, which express surface receptors for somatostatin. These scans improve the sensitivity of conventional radiology when tumor site identification is difficult (89). 68Gallium-SSTR-PET/CT likely offers the highest sensitivity (89). One study found that cortisol-producing adenomas had a higher average FDG-PET SUVmax of 5.9 compared to nonfunctioning masses (average SUVmax 4.2) and aldosterone-producing adenoma (SUVmax 3.2), and an SUVmax cut-off of 5.33 had 50.0% sensitivity and 81.8% specificity in localizing a cortisol-producing adenoma (90). Thus, FDG-PET may aid in the characterization and prioritization of adrenocortical nodules for surgery, particularly in the setting of bilateral adrenocortical masses.
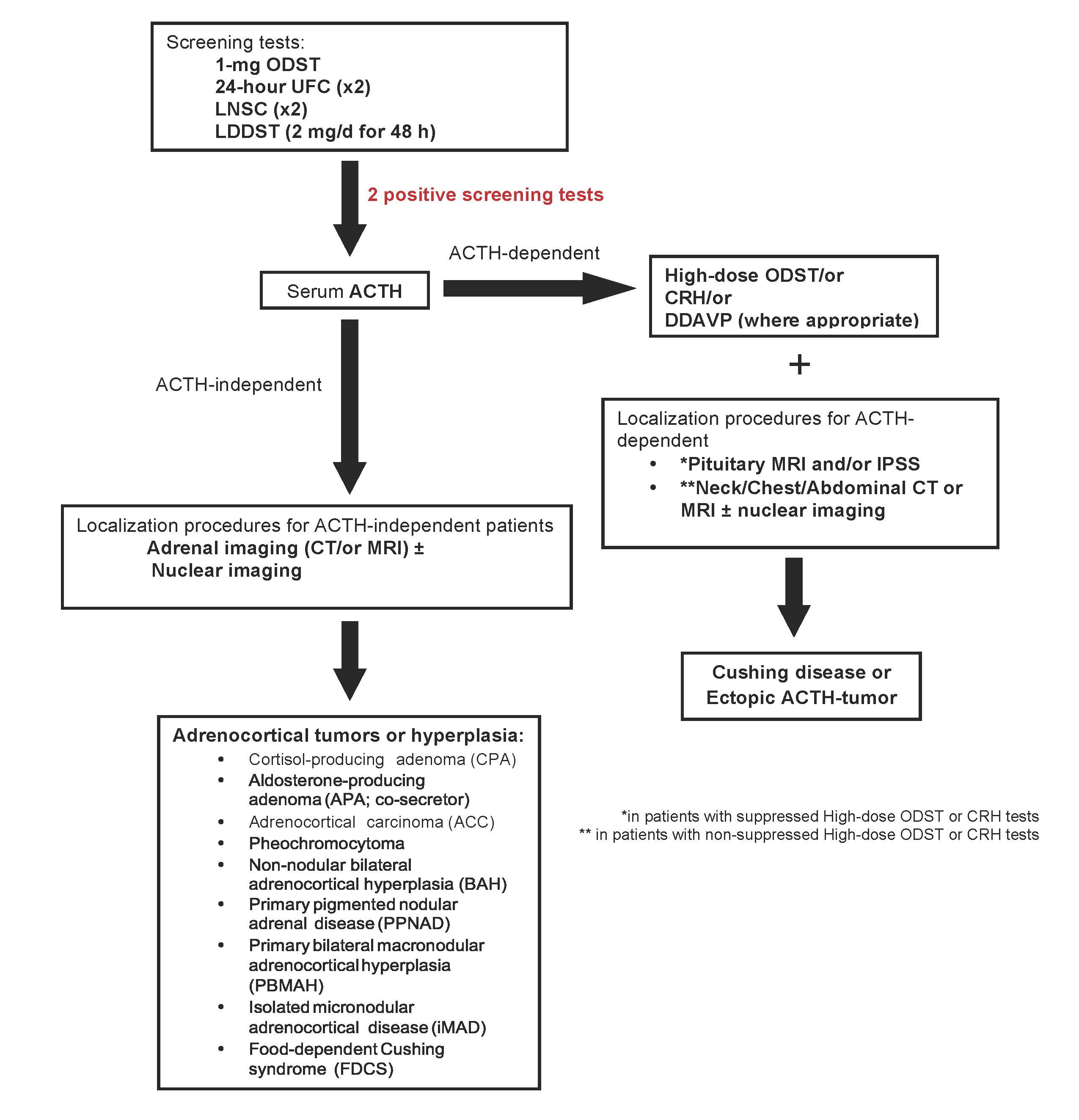
Figure 2. General diagnostic approach of Cushing syndrome (62, 65, 91)
Primary Aldosteronism (PA)
Primary aldosteronism (PA) refers to a group of disorders that produce aldosterone in an unregulated fashion, leading to HTN, sodium retention, suppression of plasma renin, and increased potassium excretion (± hypokalemia) (24, 92). The causes of PA are bilateral nodular or non-nodular adrenocortical hyperplasia (BAH; ~60%), aldosterone-producing adenoma (APA; ~30%), familial hyperaldosteronism (FH; ~2-6%), primary bilateral macronodular adrenocortical hyperplasia (PBMAH; <1%), adrenocortical carcinoma (ACC; < 1%), and ectopic aldosterone production (extremely rare). PA due to an aldosterone-producing adenoma is also known as Conn’s syndrome, in recognition of Dr Jerome Conn (93).
The 2016 Endocrine Society Clinical Practice Guidelines (24) recognizes PA as a public health issue (94), advocating for universal screening. This is particularly important, as the incidence of PA is likely higher than traditional assumptions, estimated to affect >10% of hypertensives, both in general and in specialty settings (24, 95-98). The current guidelines have modified their screening approach, to recommend screening ~50% (99) of patients with HTN (see Table 7).
Table 7. Patients at Increased Risk of Developing Primary Aldosteronism
|
· Sustained BP >150/100 mm Hg on each of three measurements obtained on different days · Hypertension (>140/90 mm Hg) resistant to three conventional antihypertensive drugs (including a diuretic) · Controlled BP (<140/90 mm Hg) on four or more antihypertensive drugs · Hypertension and spontaneous or diuretic-induced hypokalemia · Hypertension and adrenal incidentaloma* · Hypertension and obstructive sleep apnea syndrome · Hypertension and a family history of early onset hypertension · Hypertension and cerebrovascular accident at a young age (<40 years) · All hypertensive first-degree relatives of patients with primary aldosteronism
|
*An asymptomatic adrenal mass detected on imaging not performed for suspected adrenal disease
Screening tests for Primary Aldosteronism:
The following factors should be considered before screening patients for PA. Medications, advanced age, dietary sodium/potassium, and hypokalemia may affect the screening tests. Presence of kidney disease, RVH, malignant HTN or pregnancy should be ruled out to avoid false-positives or negatives. In pregnancy, increases in plasma progesterone or other steroids competitively inhibit the effects of excess aldosterone on its receptor, and may cause remission of PA (100). Lack of uniformity in screening criteria exist due to variability in screening protocols, assay methods, and individual factors, including medication use, age, sex, and presence of kidney disease (24). Although HTN and hypokalemia are suggestive of PA, the majority of patients are normokalemic (24, 98). However, an uncorrected hypokalemia may lead to a false negative screen for PA.
PA is screened with the plasma aldosterone concentration (PAC) to plasma renin activity (PRA) ratio (ARR). ARR is an easy, inexpensive, rapid and accurate means of screening for PA (96). After the patient has been up for at least 2 hours and seated for 5 (up to 15) minutes, a midmorning sample for PAC and PRA is collected and maintained at room temperature. The best correlation between PRA and PAC is achieved with low sodium intake, while the patient is in upright position. Although substantial variability in cutoff values for ARR exists, a minimum PAC of 15 ng/dL (410 pmol/L) and PRA of <1 ng/mL/h are used as screening criteria, with the most commonly adopted cutoff values for ARR is 30 (in conventional units; 90% sensitivity and 91% specificity). In the 2016 Guidelines (24), the need for further confirmatory testing in the setting of spontaneous hypokalemia, PRA below detection levels plus PAC >20 ng/dL (550 pmol/L), is not needed. However, ~ 36% of patients with PA have a PAC <15 ng/dL (101).
PAC and PRA are influenced by salt intake. Disproportionate elevation of PAC in relation to 24-hour urinary sodium excretion is usually seen in patients with PA. Suppressed PRA, for the level of the preceding day 24-hour urinary sodium excretion, is suggestive of PA. Individuals with PA tend to have a higher BP and lower serum potassium levels while on a high-sodium diet. A sodium restriction neutralized these differences (102). Thus, optimal screening for PA should occur under conditions of high sodium, as sodium restriction can significantly raise PRA, normalize ARR, and result in false interpretation of PA screening, particularly in the milder phenotypes of PA, where PRA is not as suppressed (103). It is important to note that a suppressed PRA does not differentiate between PA and low renin essential HTN or other secondary forms of HTN. In addition, other diseases such as excess mineralocorticoids other than aldosterone, apparent mineralocorticoid excess, and Liddle syndrome are associated with suppression of PRA.
There are situations where ARR may produce false negative or positive results. A quarter of patients with essential HTN have low or suppressed PRA, which may affect the interpretation of ARR. Drugs that interfere with renin or aldosterone measurements should be stopped at least two weeks prior to screening. The most common drugs that should be avoided during the screening process are: adrenergic blockers, central α-2 agonists (e.g.: clonidine and α-methyldopa), NSAIDs, K-wasting diuretics, K-sparing diuretics, ACEi, ARBs, dihydropyridine calcium channel blockers, and renin inhibitors. Drugs that have no/or limited influence on ARR are α-adrenergic blocker (prazosin, doxazosin, terazosin), hydralazine, verapamil, fosinopril, and atenolol. Mineralocorticoid antagonists (e.g. eplerenone and spironolactone) raise PAC and PRA, and should be withdrawn for at least 4-6 weeks, or longer, prior to testing (104). β-blockers lower PRA (105, 106) and produce false positive results (104). Calcium channel blockers could lower PAC, increase PRA (107), and produce false negative results (104), masking the diagnosis of PA (108). Similarly, ACEi and ARBs could produce false negative results through raising PRA (104, 109). Alpha-blockers and α-methyldopa when used for a short time during the work up of PA may not affect screening. The use of statin therapy among hypertensive and diabetic subjects was associated with lower aldosterone secretion in response to angiotensin II and a low-sodium diet (110). The 2016 Endocrine Society Clinical Practice Guidelines on PA (24) advocate for testing with interfering medications particularly in severe cases (111), to avoid delay in diagnosis, with the caveat that testing should be repeated if the results are inconclusive or difficult to interpret.
The lower limit of detection varies among different PRA assays and can have a dramatic effect on ARR. Since ARR is highly dependent on plasma renin, false positive PRA could be expected when PAC is low. Although PRA is convenient for estimating the biological activity of the renin system, it does not necessarily reflect its actual concentration. A direct renin concentration (DRC; mU/L, conversion factor from PRA to DRC is 12) is an alternative test that can confirm a low renin state. This could be particularly useful in low-renin HTN, as observed in African Americans (112). DRC and PRA are poorly correlated in the range where PRA is <1 ng/mL/h.
The post captopril ARR enhances the accuracy for diagnosing PA (98). A ratio greater than 35 has sensitivity and specificity of 100% and 67-91%, respectively, compared with 95.4% and 28.3%, respectively, at baseline in patients with PA (98). This test appears to be as sensitive as salt loading in confirming a diagnosis of PA (113). This test is performed by administering 25 mg of captopril orally, taken 2 hours before sampling (114).
Recently, a new overnight diagnostic test was developed to simplify screening for PA. The test consisted of the fludrocortisone-dexamethasone suppression test (FDST) and the new overnight diagnostic test (DCVT) using a combination of dexamethasone, captopril and valsartan (115). The estimated sensitivity and specificity were 91 and 100%, respectively, for the post-FDST ARR, whereas 98% and 89% and 100% and 82% for the post-DCVT ARR and post-DCVT autonomous aldosterone secretion, respectively, with selected cutoffs of 0.32 and 3 ng/dL, respectively (115). The diagnosis of PA was confirmed in 44/45 (98%) using this non-laborious approach.
Prior evidence from observational and intervention studies suggested a modifiable relationship between the renin-angiotensin-aldosterone system (RAAS) and parathyroid hormone levels in humans (116). Recent evidence suggests that higher serum aldosterone concentration is associated with higher serum parathyroid hormone concentration, which is decreased with the use of RAAS inhibitors (117). This relationship does not alter the current screening recommendations for PA.
Confirmatory Tests for Primary Aldosteronism:
The 2016 Endocrine Society Clinical Practice Guidelines on PA (24) recommends that patients with a positive ARR undergo one or more confirmatory tests to definitively confirm or exclude the diagnosis of PA. However, in the setting of spontaneous hypokalemia, PRA below detection levels, and PAC >20 ng/dL (550 pmol/L), proceeding directly to subtype classification with confirmatory testing is warranted. The following tests are used to confirm PA:
Oral sodium loading test - 24h urinary excretion of aldosterone is measured after 3 days of high salt intake (>200 meq/day, ~ 6 g/day). 24 hour urinary sodium and creatinine should be measured simultaneously to ensure high sodium intake and adequacy of urine collection. Failure of high salt to suppress urinary aldosterone excretion to <11 µg/24 hours is diagnostic for PA. This test has a sensitivity of 96% and specificity of 93% for PA (113, 118).
Fludrocortisone suppression test - This test is performed by administering fludrocortisone 0.1 mg orally every 6 hours or 0.2 mg orally every 12 hours and sodium chloride >200 mmol orally/day for 4 days. Failure to suppress upright PAC to <5 ng/dL by day 4 confirms the diagnosis of PA. Upright PRA should be suppressed to <1 ng/ml/h on day 4 of the test. Since hypokalemia inhibits aldosterone secretion, potassium chloride supplement should be given to keep plasma potassium levels close to or in the normal range. This test is considered the most sensitive test to diagnose PA.
Saline suppression test - This test is performed by measuring PAC in the supine position after intravenous administration of 500 mL/hour of 0.9% sodium chloride for 4 hours. Failure to suppress PAC <10 ng/dL at the end of this test confirms the diagnosis of PA (119, 120). This test is easy to perform on an outpatient basis.
Both the fludrocortisone and saline suppression tests are contraindicated in patients with severe HTN, congestive heart failure, advanced kidney disease, cardiac arrhythmia, or severe hypokalemia.
Captopril challenge test - This test is performed by administering 25-50 mg captopril orally in the seated or standing position for at least 1 hour. PAC and PRA are measured before and 1 hour after administration of captopril. If PAC >12 ng/dL or ARR >26, the test is considered positive (121).
Differentiating Between Aldosterone-Producing Adenoma (APA) and Bilateral Adrenal Hyperplasia (BAH)
Changes in PAC on upright posture - Patients with APA show no change or reduction in PAC on upright posture, unlike patients with BAH. This test is performed by measuring PAC in the supine position and after 4 hours of upright posture. ~ 70% of patients with BAH respond by increasing PAC by at least 50%.
Bilateral adrenal venous sampling (AVS) - When surgical treatment is feasible and desired by the patient, an experienced radiologist should use AVS to differentiate between APA and BAH (24). Younger patients (<35 years old) with spontaneous hypokalemia, marked aldosterone excess, and unilateral adrenocortical lesions may not need AVS before proceeding to unilateral adrenalectomy (24).
PAC and cortisol levels are measured in the inferior vena cava (IVC) and right and adrenal veins before and serially after intravenous injection of synthetic ACTH, cosyntropin 0.25 mg. ACTH stimulation improves cortisol gradients and aldosterone secretion, resulting in a reduction in the proportion of nondiagnostic studies (122). Moreover, ACTH stimulation significantly reduces bilateral aldosterone suppression (aldosterone/cortisol (A/C) ratios in the adrenal veins are bilaterally lower than that in the inferior vena cava) with a single AVS procedure (123). The purpose of measuring plasma cortisol is to confirm the site of the sampling catheter, by correcting for differences in dilution of adrenal with non-adrenal venous blood when assessing for lateralization. Plasma cortisol levels are much higher in adrenal veins than IVC. However, simultaneous autonomous overproduction of cortisol and aldosterone is increasingly recognized, particularly in BAH, and unilateral cortisol overproduction with contralateral suppression could confound the interpretation of AVS results. Thus, measuring plasma free metanephrine during AVS to calculate lateralization ratios may circumvent this problem (124, 125). Basal combined ratio during AVS carries the best sensitivity for the detection of AVS selectivity at all cutoff values (126).ACTH stimulation acutely stimulates aldosterone secretion and will help magnify the differences in PAC levels between the two adrenal glands. The A/C ratio of the involved to contralateral side provides the best diagnostic accuracy for determining if one adrenal is responsible for increased aldosterone production. With determination of bilateral selective samples, ratios (A/C on involved side)/(A/C of IVC) ≥1.1, or of (A/C involved side)/(A/C opposite side) ≥2 provide the best compromise of sensitivity and false positive rates for lateralization of the etiology of PA (127). Contralateral suppression, defined as A/C (adrenal) ≤ A/C (peripheral) on the unaffected side, combined with a ratio ≥2 times peripheral on the affected side, correlates with good BP and biochemical outcomes from surgery, and could be used as a factor in deciding whether to offer surgery for treatment of PA (128). Moreover, in patients with PA, where the lateralization index is <4 on AVS, contralateral suppression of aldosterone is an accurate predictor of a unilateral source of aldosterone excess (129). Basal aldosterone contralateral suppression could predict residual hyperplasia and post-operative outcomes (130).In patients without the right AVS due to issues related to canulation or nonselective, a multinomial regression modeling can detect lateralization of aldosterone secretion in most patients and could eliminate the need for repeat AVS (131).
Current evidence supports the use of LC-MS/MS-based steroid profiling during AVS to achieve higher aldosterone lateralization ratios in patients with APAs than immunoassay (132). Moreover, LC-MS/MS enables multiple measures for discriminating unilateral from bilateral aldosterone excess, with potential use of peripheral plasma for subtype classification (132).
Unilateral adrenalectomy is beneficial in patients with a unilateral source of hyperaldosteronism and/or in some patients with apparent bilateral PA (133). Patients with PA that undergo unilateral adrenalectomy enjoy a higher quality of life scores than their medically treated counterparts (134). To identify the likelihood of complete resolution of HTN without further need of lifelong antihypertensive therapy following unilateral adrenalectomy, the Aldosteronoma Resolution Score could be calculated (135). This score accurately identifies individuals at low (≤1) or high (≥4) likelihood of complete resolution of HTN, based on four readily available predictors (2 or fewer antihypertensive medications, BMI ≤25 kg/m2, duration of HTN ≤6 years, and female sex) (135), and can help clinicians objectively inform patients of likely clinical outcomes before surgical intervention.
In 2014, a consensus was reached on several key issues in relation to AVS, including the selection and preparation of the patients, the procedure for its optimal performance, and the interpretation of its results for diagnostic purposes even in the most challenging cases (136). A recent study demonstrated that treatment of PA based on CT or AVS subtype classification did not show significant differences in intensity of antihypertensive medication or clinical benefits for patients after 1 year of follow-up, which challenges the current recommendation to perform AVS in all patients with PA (137).
Imaging Modalities Useful in the Evaluation of Adrenocortical Masses:
Ultrasonography - Although simple and economic, this imaging modality has a lower sensitivity in detecting adrenocortical masses than CT or MRI (138, 139). The sensitivity varies with the extent of the adrenocortical mass (65% for mass <3 cm, and up to 100% if >3 cm) (140). The role of ultrasonography in differentiating benign from malignant adrenocortical masses is limited (141).
Adrenocortical scintigraphy - This modality uses cholesterol based radioactive tracers and include 131iodine 6-β-iodomethylnorcholesterol (NP-59) and 75selenium-Se-6-selenomethyl-19-Norcholesterol (142). Concordant and discordant patterns of uptake may not be differentiated in lesions <2.0 cm in diameter (143, 144). Sensitivity (71%-100%) and specificity (50%-100%) range widely for differentiating benign from malignant tumors (143, 145).
CT and MRI - These conventional imaging modalities assist in the subtyping of the etiology of PA. High resolution CT and MRI of the adrenal glands have poor sensitivity in localizing small APAs (<5mm in diameter) (127, 146). CT of the adrenal glands analyzes contiguous 2–5 mm-thick CT slices on multiple sections using multidetector row protocols (147). CT and MRI can help determine whether an adrenocortical mass is an adrenocortical carcinoma and can also assess for local tumor invasion and metastatic disease (148, 149). A CT cut-off at 4.0 cm has a sensitivity of 93% (150), while an unenhanced CT density of ≤10 HU has a sensitivity of 96–100% and a specificity of 50–100% in differentiating benign from malignant tumors (151-155).
A systematic review of diagnostic procedures to differentiate unilateral from bilateral adrenocortical lesions in PA has found that CT/MRI misdiagnosed 37.8% of patients when diagnostic accuracy of AVS was used as a main criterion for diagnosing laterality of aldosterone secretion, suggesting that these imaging modalities may not be sufficient for a definitive diagnosis of PA (156). Enhanced CT assists in distinguishing between lesions that are lipid-rich (aldosterone-producing adenoma, cortisol-producing adenoma) and lipid-poor (eg: pheochromocytoma, adrenocortical carcinoma). Lipid-rich adenomas “washout’’ contrast faster. They can be differentiated by attenuation values or the percentage or relative percentage of washout as early as 5-15 min after enhancement if the unenhanced CT density is >10 HU (153). Lipid-rich and lipid-poor lesions have a relative percentage washout on delayed scans of >50% and <50%, respectively (157). One study demonstrated a washout value of 51% at 5 min and 70% at 15 min in benign lesions, with a sensitivity and specificity for the diagnosis of adrenocortical adenoma of ~ 96% at a threshold attenuation value of 37 HU on the 15-min delayed enhanced scan (153). MRI is as accurate in distinguishing lesions that are lipid-rich from lipid-poor. Chemical shift imaging MRI can sort out lipid-rich lesions with a sensitivity of 84–100% and a specificity of 92–100% (149, 158-160). Adenomas appear as hypo- or iso-intense on T1-weighted images, and hyper- or iso-intense on T2-weighted images (161). Combining adrenal imaging and AVS, the effective surgical cure rate for PA is 95.5%, with a poor (58.6%) accuracy of CT and MRI in detecting unilateral adrenal disease, although the performance was well in patients <35 years old (162).
18F-FDG PET - This modality has a sensitivity of 93-100%, and specificity of 80-100% in identifying malignant masses in the adrenal glands or elsewhere (163-167). However, some primary malignant tumors (necrotic, hemorrhagic) or those that are metastatic (>1 cm) may show a lower FDG uptake than the liver, leading to false-negatives (163, 164, 167). One study found that APAs had a SUVmax 3.2 (67), which may aid in the characterization and prioritization of adrenocortical nodules for surgery, particularly in the setting of bilateral adrenocortical masses.
PET-CT - This modality has a sensitivity of 98.5%-100%, and specificity of 92%-93.8% in detecting and differentiating between the various types of adrenocortical masses. When enhanced CT is added, the specificity is reached to 100% (168).
11C-metomidate PET- Metomidate-based tracers (bound to adrenal CYP11B enzymes) have been introduced in clinical practice recently. These techniques provide good visualization of adrenocortical lesions. This new investigation has been considered promising in differentiating between lesions of adrenocortical and non-adrenocortical origins (169, 170). 11C-metomidate PET-CT demonstrates a good sensitivity and specificity in the detection of APA (171, 172). Based on SUVmax, the specificity was as much as 100% (172). Therefore, 11C-metomidate PET-CT could be a useful noninvasive and rapid investigation to AVS in patients with adrenocortical tumors (153, (172), although this technique has low selectivity for CYP11B2 over CYP11B1.
18F-CDP2230 - This recently described modality combines nuclear imaging with a new agent that has a high selectivity for CYP11B2 over CYP11B1 with a favorable biodistribution for imaging CYP11B2 (173). 18F-CDP2230 could be a promising imaging agent for detecting unilateral subtypes of PA.
Familial Hyperaldosteronism (FH)
Familial aldosteronism (FH) represent a group of autosomal dominant (AD) disorders that is estimated to affect ~ 2-6% of all patients with PA. FH is classified into three major subtypes:
FH-I, also known as Glucocorticoid-Remediable Aldosteronism (GRA) is an AD disorder characterized by a chimeric fusion of CYP11B2 and CYP11B1 (8q24.3), rendering the aldosterone synthase hybrid gene to be under the regulation of ACTH rather than the renin-angiotensin system (174, 175). This rare monogenic form of HTN in humans with no gender predilection accounts for ~1% of PA. Increased production of aldosterone and hybrid steroids, such as 18-oxocortisol and 18-hydroxycortisol, which is suppressible to dexamethasone, is seen in GRA. Significant phenotypic and biochemical heterogeneity exist (176); males tend to have more severe HTN, and likely related to the degree of hybrid gene-induced aldosterone overproduction (177), while others may never develop HTN. Some patients may develop benign adrenocortical tumors (178). GRA should be suspected in patients with early-onset HTN (<20 years) in the setting of a suppressed PRA, a family history of PA, or early cerebral hemorrhage (<40 years) from intracranial aneurysms or hemorrhagic strokes (179).
FH-II (7p22) represents the most common form of FH that typically affects adults. FH-II is characterized by PA due to BAH, APA, or both, which is not glucocorticoid remediable (180). FH-II is clinically indistinguishable from sporadic PA. The mutations that cause FH-II are unknown, but linkage analysis has mapped them to chromosome 7p22 (181-185).
FH-III is due to a gain-of-function heterozygous germline mutation in KCNJ5 (11q2) that increase constitutive and angiotensin II-induced aldosterone synthesis. FH-III presents earlier, in childhood, with severe HTN and metabolic derangements. In FH-III, KCNJ5 is aberrantly co-expressed with CYP11B2 and in some cells with CYP11B1, which likely explains the abnormally high secretion rate of the hybrid steroid, 18-oxocortisol (186).
Recently, germline mutations in ARMC5 (16p11.2) have been implicated in PA. One study identified germline mutations across the entire ARMC5 gene in 39.3% of patients with APA (187). In addition to the germline mutations, a second somatic variant was required in AMRC5 to mediate tumorigenesis leading to polyclonal adrenocortical nodularity (60, 61, 188). Interestingly, all mutant APA’ s affected patients of African Americans decent (187), which may explain their increased predisposition to PA and/or HTN. These findings suggest that ARMC5 plays an important role in the development of APA or other adrenocortical tumors, and may represent a new subtype of FH.
Recently, germline mutations in CACNA1D, which codes for an L-type calcium channel, have been found in two cases with a syndrome of PA, seizures, and neurologic abnormalities (189, 190). A recent exome sequencing study identified a recurrent gain of function germline mutation in CACNA1H (a T-type calcium channel), in 5 unrelated families with early-onset PA and HTN (189, 191). These findings suggest that mutations in calcium channels play an important role in the development of PA, and may represent a new subtype of FH.
The 2016 Endocrine Society Clinical Practice Guidelines (24) recommends screening for GRA in patients diagnosed with PA and:
- Onset of HTN <20 years of age
- A family history of PA
- Strokes or other early cerebrovascular complications at <40 years of age
Screening could begin at puberty and then at every 5 years interval but its utility needs further confirmation. Most patients with a clear diagnosis of GRA are severely hypertensive (177, 192). The biochemical profile of individuals with GRA is represented in Table 8.
Table 8. Laboratory confirmation of Glucocorticoid-Remediable Aldosteronism
|
|
Disease | Laboratory profile |
GRA |
· Hypokalemia (not always present), high urinary potassium · Suppressed PRA · PAC or urinary aldosterone is normal or mildly elevated · ARR >30. Plasma aldosterone fails to rise or falls during 2 hour of upright posture following overnight recumbency (193, 194) · Elevated 24 hour urinary and plasma levels of 18-oxocortisol and 18-hydroxycortisol (195). Increased urinary 18-hydroxycortisol/total cortisol metabolites ratio. 18-oxocortisol is 20-30 higher in GRA than APA (196, 197) · PAC <4 ng/dL after suppression with dexamethasone 0.5 mg PO every 6h for 2-4 days (LDDST) is diagnostic for GRA (197). However, some patients may fail to suppress. Aldosterone is markedly elevated in response to ACTH administration (198-200). Other studies have found some patients with PA but without the chimeric gene and suppression of PAC with dexamethasone treatment so that this test may be interpreted with caution in patients with possible GRA (201) · Genetic testing using long PCR-based methods for detecting the hybrid GRA gene (11β-hydroxylase gene/aldosterone synthase gene) is the gold standard test for diagnosis (100% sensitivity and specificity) (174, 202)
|
Some considerations have to be made regarding the biochemical profile of GRA:
PRA level is non-specific since ~20% of patients with essential HTN have a low or suppressed renin
The degree of HTN, hypokalemia, urinary 18-oxocortisol and 18-hydroxycortisol, suppressed PRA or elevated PAC cannot be used to identify patients with GRA as they lack specificity (occurring in the other subtypes of PA) (132)
The 1 mg ODST may result in false positives while the LDDST test could produce false negative results
Dexamethasone may also suppress aldosterone in patients with APA. However, since aldosterone secretion is autonomous, DST fails to suppress to very low levels
Although LDDST is highly sensitive and specific (>90%) for GRA, some patients may show initial suppression only to rise again by day 4 of treatment or fail to suppress PAC to <4 ng/dL (197)
The major drawback of LDDST is the need for multiple blood tests, requiring either hospitalization or repeated outpatient visits. Also, LDDST is difficult to perform in children
Genetic testing could be used as a screening test for newborns of affected parents. Placental tissue or cord blood could be used. A negative test eliminates the possibility of GRA diagnosis
The LDDST and elevated 24-hour urinary and plasma levels of 18-oxocortisol and 18-hydroxycortisol could be used to diagnose GRA with the caveat that APA and BAH may result in elevated values.
Modern diagnosis of GRA relies on identification of the CYP11B1/CYP11B2 chimeric gene
Pheochromocytoma And Paraganglioma (PPGL)
PPGLs are neuroendocrine tumors that arise from adrenal (~85%, pheochromocytoma) or extra-adrenal (~15%, paraganglioma) chromaffin cells (203). These cells continuously produce and release, in an unregulated fashion, metanephrine and/or normetanephrine (“metanephrines”) from epinephrine and norepinephrine (“catecholamines”), respectively (204). The most frequent location of PPGLs is in the adrenal glands (150). The prevalence of PPGL in patients with HTN is ~0.3% (26). Testing for PPGLs should be performed in the following circumstances (205, 206):
- Signs and symptoms of PPGL, in particular if paroxysmal and/or provoked by certain medications such as glucocorticoids
- Adrenal incidentaloma, with or without HTN
- Hereditary predisposition or syndromic features suggesting hereditary PPGL (MEN1, MEN2, VHL, NF1, SDHx (203, 207-209))
- Previous history of PPGL
Sympathetic paragangliomas that arise from the sympathetic paravertebral ganglia of thorax, abdomen, and pelvis produce catecholamines and are rarely silent. Parasympathetic paragangliomas that arise from the glossopharyngeal and vagal nerves in the neck and at the base of the skull head and neck do not produce catecholamines, but rather dopamine (206, 210, 211). The classic triad of headaches 71%, sweating 65%, palpitations 65% and HTN has a 91% sensitivity, and 94% specificity, in diagnosing PPGL, although only seen in <30% of cases (212). Other symptoms that may point to the diagnosis of PPGLs are orthostatic hypotension (head and neck tumors that secrete dopamine) (10-50%), hyperglycemia (40%), weight loss (20-40%), flushing (10-20%), constipation, and pallor. Symptoms may be exacerbated by activity depending on the location of the PPGL, including urination (bladder PPGL), sexual intercourse or exercise (206, 212). Asymptomatic individuals with PPGL have been reported by various studies (206, 213). Normotensive PPGLs exist, and are often times identified as adrenal incidentalomas (incidence ~5%; 43% of patients had HTN) (150, 213). Patients with such tumors may not have elevated plasma or urinary fractionated metanephrines and may not need preoperative alpha blockade (214).
Biochemical screening for PPGL:
Figure 3 represents a general screening algorithm for PPGL. Measurement of plasma free or urinary fractionated metanephrines (“fractionated metanephrines”) by LC-MS/MS is the most reliable and specific screening test for the diagnosis PPGLs (215-217). This assumes that the reference intervals have been appropriately established and measurement methods are accurate and precise (206, 215). Since the concentrations of normetanephrine sulfate and metanephrine-sulfate in plasma are about 20-30 fold higher than the levels of their free metabolites, the measurements of their deconjugated metabolites in plasma provides major advantages over their traditional measurements (218). Plasma free metanephrines reflect direct production by the tumor tissue and are considered the best test for excluding or confirming pheochromocytoma (204). Free metanephrine production is continuous and independent of catecholamine release (166). Thus, measurement of plasma free metanephrines is more reflective of the tumor production than catecholamines and normetanephrine levels.
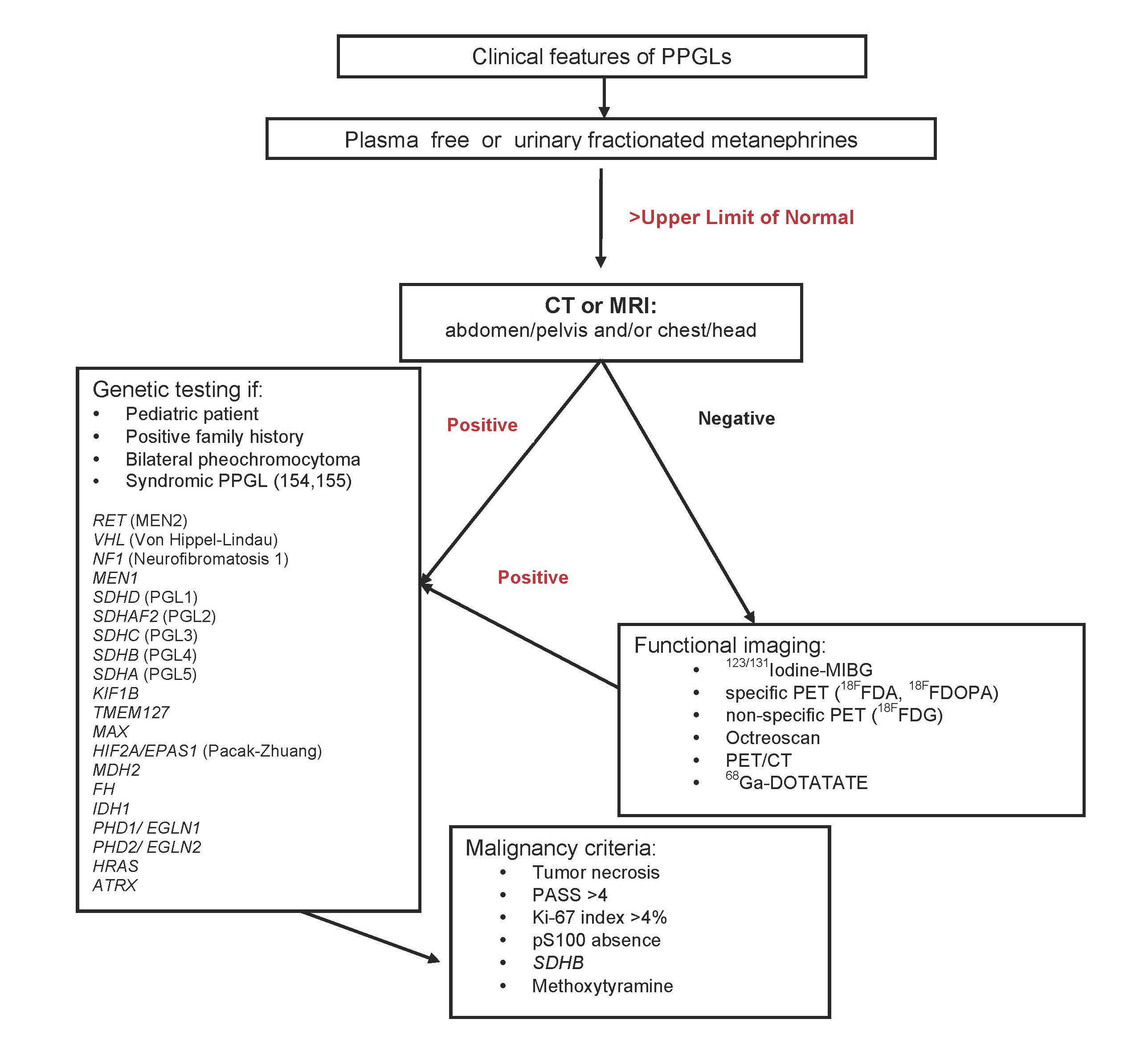
Figure 3. Flow chart for diagnostic evaluation for Pheochromocytoma. Adapted after: Waguespack SG et al. (219). The criteria of malignancy is adapted from de Wailly et al. (211).
A single collection of plasma free metanephrines (sensitivity 99%, specificity 89%) or urinary fractionated metanephrines (sensitivity 97%, specificity 69%) is equally recommended for screening, and combination of tests offers no advantage (206, 215, 217, 220). If urinary fractionated metanephrines are favored, then measurement of urinary creatinine for verification of collection should be performed. Fractionated metanephrines are superior to plasma catecholamines (sensitivity 84%, specificity 81%), urinary catecholamines (sensitivity 86%, specificity 88%) or urinary vanillylmandelic acid (sensitivity 64%, specificity 95%) (206, 217) (see Table 9). A spot urine sample should not be used for screening (206). Metanephrines can be detected in saliva with LC-MS/MS with sufficient sensitivity and precision but are not currently validated for screening (221). To avoid false positives, caffeine, smoking and alcohol intake should be withheld for 24 hours prior to testing, and the blood sample should be drawn in lavender or green-top tube, transferred on ice, and then stored at -80°C until analyzed (206, 215). During testing, the patient should not be ill or under significant stress or strenuous physical activity.
Table 9. Sensitivity and specificity of biochemical tests for diagnosis of pheochromocytoma and paraganglioma (217)
|
||
Test | Sensitivity (%) | Specificity (%) |
Plasma free metanephrines | 99 | 89 |
Plasma catecholamines | 84 | 81 |
Urine fractionated metanephrines | 97 | 69 |
Urine catecholamines | 86 | 88 |
Urine total metanephrines | 77 | 93 |
Urine vanillylmandelic acid | 64 | 95 |
The highest diagnostic sensitivity for plasma free metanephrines is reached if the collection is performed in the supine position after an overnight fast and while the patient is recumbent in a quiet room for at least 20-30 minutes, and interpreted using the upper limit of the age-adjusted reference interval (206, 215, 222). If supine fasting sampling is performed, then the majority of patients with PPGLs can be recognized (215, 223). In centers where the supine position is not possible, then urinary fractionated metanephrines should be used for screening. A negative test result for plasma free metanephrines while seated is as effective for ruling out PPGL as negative results while supine (222). Measurement of seated serum metanephrines above the upper limit of the seated reference range has a diagnostic sensitivity and specificity of 93% and 90%, respectively (224). However, this approach can lead to a 5.7-fold increase in false-positives (223), necessitating repeat sampling.
Levels of fractionated metanephrines within the reference range usually exclude PPGLs (225), while equivocal results, which is seen in ~25% of all patients with PPGLs, require additional tests. Functional PPGL in patients with MEN2 and NF1 are characterized by increases in plasma free metanephrine, indicating epinephrine production, while VHL patients have increases in normetanephrine (indicating norepinephrine production) (226). Elevations in methoxytyramine (indicating dopamine production) are seen in ~70% of patients with SDHB and SDHD mutations (226). Exceptions for false-negative fractionated metanephrines exist, including PPGLs that are <1 cm in size, those that produce only dopamine (e.g. head and neck PPGLs), or those that are biochemically silent.
True- from False-positive Elevations of Fractionated Metanephrines
Levels of fractionated metanephrines >3 fold above the upper limit of the age-adjusted reference interval are rarely false-positives (227). The clonidine-suppression test is useful in distinguishing between true- from false-positive elevations in screening tests (228): 0.3 mg/70 kg body weight of clonidine hydrochloride is given orally, and plasma normetanephrine is measured at baseline and 3-hours after administration. A decrease in levels by >40%, or below the assay’s upper reference limit, suggests sympathetic activation, with a diagnostic specificity of 100% and a sensitivity of 87% in ruling out PPGLs with borderline elevations (227). Sensitivity increases to ~95% for elevations above borderline values (227). It is important to note that this test can only be used for PPGLs that secrete either norepinephrine or normetanephrine (229). The glucagon stimulation test should not be used in clinical practice given its insufficient diagnostic sensitivity for PPGL and potential to induce crisis (206, 230). There is insufficient evidence to use urine fractionated metanephrines and serum/plasma chromogranin A in conjunction for evaluation of borderline elevations in screening tests (231, 232).
Plasma norepinephrine and dopamine can be increased up to 3-fold in patients on hemodialysis without PPGL (233). Plasma free metanephrines and catecholamines are 2-3 fold higher in patients with renal failure compared with other groups (healthy normotensives, hypertensives and patients with VHL). However, plasma free metanephrines are relatively independent of renal function and are the test of choice in the diagnosis of PPGL among patients with renal failure (233) or those in the intensive-care unit. Urinary and plasma fractionated metanephrines have the highest sensitivity to diagnose PPGL in pregnancy (234).
In preparation for screening, discontinuation of all medications and substances that could interfere with the results should be performed (235) (see Figure 4.). The following medications raise fractionated metanephrines and catecholamines: tricyclic antidepressants, selective norepinephrine reuptake inhibitors > selective serotonin reuptake inhibitors, monoamine oxidase inhibitors, cocaine, and α or β-blockers. The following medications have less or little influence on biochemical screening and can be continued during screening: selective α1-adrenoceptor blockers, diuretics, ACEi, and ARBs. The following medications may cause a direct analytical interference with the assays (not observed with LC-MS/MS), and should be withdrawn 5 days before testing: acetaminophen, labetalol, sotalol, buspirone, α-methyldopa, and 5-aminosalicylic acid (mesalamine, and its prodrug, sulfasalazine). Withdrawal from the following can markedly elevate plasma or urinary fractionated metanephrines or catecholamines: sedatives, opioids, benzodiazepines, alcohol and smoking.
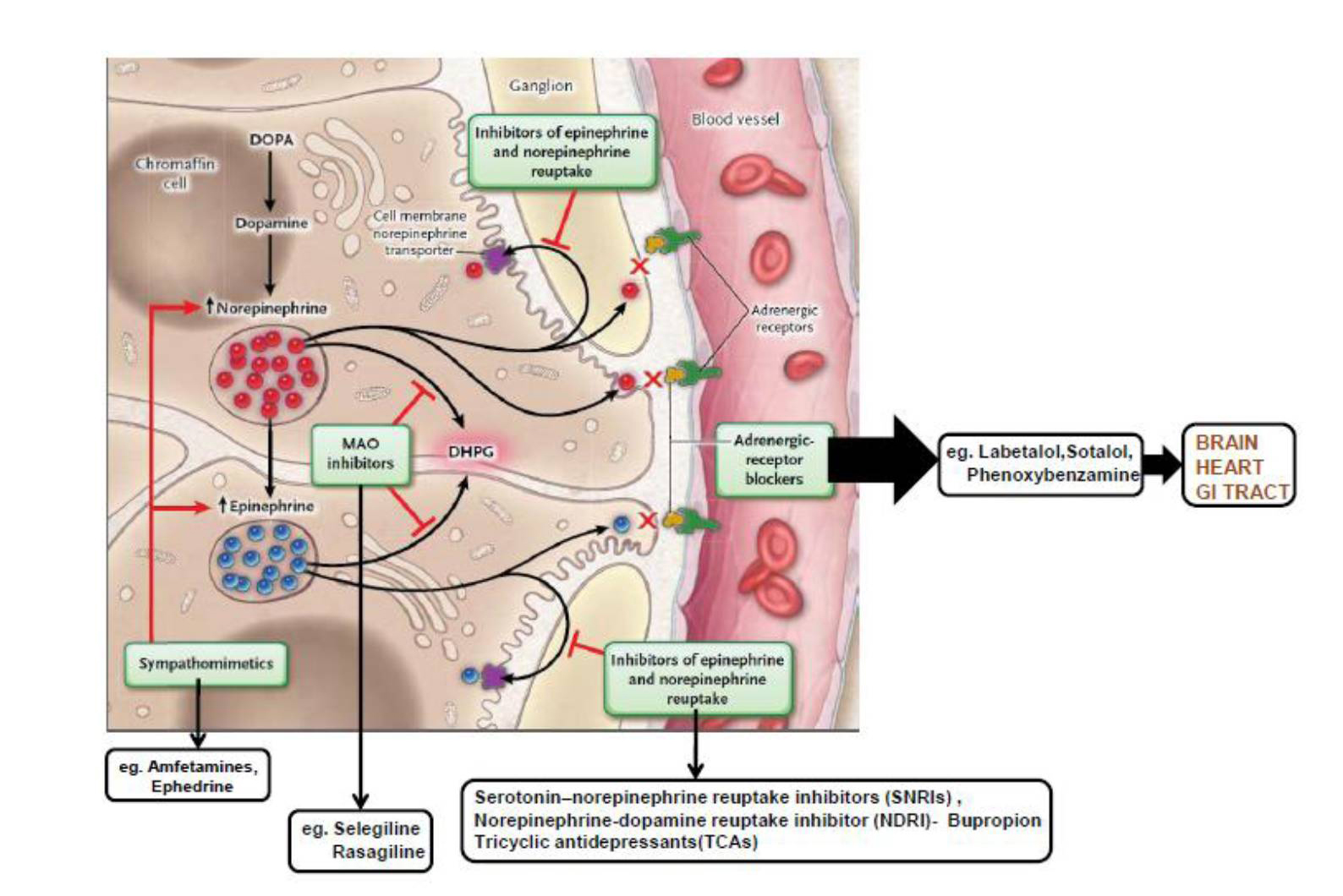
Figure 4. Mechanisms of Pharmacologic Interference with Catecholamines and Metanephrines (235). Monoamine oxidase- (MAO), dihydroxyphenylglycol- (DHPG), DOPA- dihydroxyphenylalanine. Adapted from Neary et al. (235)
Imaging Modalities useful in the Evaluation of PPGL
CT and MRI - CT with contrast is very sensitive (88-100%) for localization of PPGLs that are >5 mm in diameter (236, 237). As with MRI, CT provides excellent topographical resolution but lacks specificity. PPGLs are homogeneous or heterogeneous, necrotic with some calcifications, solid, or cystic on CT. Most (>85%) PPGLs have an unenhanced attenuation of >10 HU on CT (154), although occasionally could have >60% washout on delayed imaging (238). A high signal intensity on T2-weighted MRI, referred to as a bright signal, is characteristic of PPGLs (239). The Endocrine Society Guidelines (206) recommend a CT scan of the abdomen and pelvis as the first radiographic test in evaluating PPGL, and is the preferred initial test in detecting lung metastasis. MRI is preferred in patients with metastatic PPGLs, for detection of skull base and neck paragangliomas, in patients with surgical clips causing artifacts when using CT, in patients with an allergy to CT contrast, and in patients in whom radiation exposure should be limited (children, pregnant women, patients with known germline mutations, and those with recent excessive radiation exposure) (206).
Functional imaging - 123I-metaidobenzylguandine (123I-MIBG) has a sensitivity and specificity of ~ 85% for pheochromocytomas and ~ 70% for paragangliomas, and is recommended as a functional imaging modality in patients with metastatic PPGLs detected by other imaging modalities when radiotherapy using 131I-MIBG is planned (206). Extra-adrenal and small adrenal pheochromocytomas are more likely to be under detected by 123I-MIBG and produce false negative results (240). Preparation with sodium perchlorate or potassium iodide is necessary 2 days before and 1 week after 131I-MIBG therapy to protect the thyroid gland (241). Some drugs that interfere with MIBG uptake such as labetalol, reserpine, digoxin, ACEi, various antidepressants/antipsychotics, and some of the sympathomimetics should be withdrawn 3-10 days before treatment (242). ~50% of normal adrenal glands demonstrate physiological uptake of 123I-MIBG, which may lead to false positives (243).
18F-FDA PET/CT is the preferred technique for the evaluation of patients with metastatic PPGLs. However, 68Ga-DOTA(0)-Tyr(3)-octreotate (68Ga-DOTATATE), a PET radiopharmaceutical with both high and selective affinity for somatostatin receptors, showed the highest lesion-based detection rate of 97.6 % when compared to other modalities in the localication of sporadic metastatic PPGLs (244, 245). 18F-FDG PET/CT, 18F-FDOPA PET/CT, 18F-FDA PET/CT, and CT/MRI showed detection rates of 49.2 %, 74.8 %, 77.7 %, and 81.6 %, respectively (244). Moreover, 68Ga-DOTATATE PET/CT was found to be the most sensitive technique in the detection of head and neck PPGLs, especially those that harbor mutations in SDHD, which may be very small and fail to concentrate sufficient 18F-FDOPA (246). Gene-targeted radiotherapeutics and nanobodies-based theranostic approaches are the future of imaging in PPGLs (247).
RARE CAUSES OF ENDOCRINE HYPERTENSION
Congenital Adrenal Hyperplasia: 11ß-Hydroxylase Deficiency
11β-hydroxylase deficiency is the second commonest variant of CAH (after 21-hydroxylase deficiency) with an incidence of 1 in 100,000–200,000 live births (248). This condition is caused by mutations in the 11β-hydroxylase gene (CYP11B1) and is inherited in an autosomal recessive (AR) manner. The highest prevalence is seen in Moroccan Jews, with an approximate incidence of 1 in 5000–7000 live births (249). A defective CYP11B1 enzyme leads to HTN from elevated deoxycorticosterone (DOC) and possibly other steroid precursors, and hyperandrogenism from shunted precursors into the androgen synthesis pathway (250). The classic form presents as virilization of the external genitalia in 46,XX newborn females, and precocious pseudopuberty in both sexes. The nonclassic form presents with hyperandrogenism during childhood. 11ß-hydroxylase deficiency is caused by several mutations in the CYP11B1 gene. Patients do not present with adrenal insufficiency due to the glucocorticoid effects of excess corticosterone. Biochemical profile includes variable hypokalemia, variable hyporeninemia, ↓↓ aldosterone, ↓cortisol, ↑ACTH, ↑11-deoxycortisol, ↑DOC, and ↑ 19-nor-DOC. Other laboratory abnormalities include elevated serum level of 17-hydroxyprogesterone (17-OHP), androstenedione and urinary pregnanetriol. See Tables 9-11 for laboratory tests and ratios provided by questdiagnostics.com.
Congenital Adrenal Hyperplasia: 17α-Hydroxylase Deficiency
17α-hydroxylase deficiency is an AR condition that results from a defective CYP17A1 (248, 251, 252). This enzyme is responsible for catalyzing both 17-hydroxylase and 17,20 lyase activity. Thus, this condition results in glucocorticoid and sex steroid deficiency, and impairs both adrenal and gonadal function. Certain ethnicities are at higher risk of 17α-hydroxylase deficiency, including Canadian Mennonites and Dutch Frieslanders, suggesting a founder effect (253). Biochemical features are ↑DOC, ↓11-deoxycortisol, ↓↓ aldosterone, ↓renin , ↓K, ↓plasma 17-OHP, and ↓testosterone. The accumulation of the mineralocorticoid precursors corticosterone and DOC exert glucocorticoid and mineralocorticoid activity respectively and lead to HTN with hypokalemia. Adrenal insufficiency is not a characteristic feature of this condition. The classic presentation of the severe form is a phenotypic female (46,XX or 46,XY) with HTN and absence of secondary sexual characteristics (254). In the partial form, 46,XY patients may present with undervirilization and present as infants with ambiguous genitalia. Diagnosis of this autosomal recessive condition is suggested by delayed puberty, absent secondary sexual characteristics or amenorrhea combined with the typical biochemical findings (254). Genetic testing for mutations in the CYP17A1 gene confirms the condition. See Tables 9-11 for laboratory tests and ratios provided by questdiagnostics.com.
Apparent Mineralocorticoid Excess
The enzyme 11β-hydroxysteroid dehydrogenase type 2 (11βHSD2) is highly expressed in the kidneys where it metabolizes cortisol to cortisone to prevent the mineralocorticoid receptor (MCR) from inappropriate activation by cortisol (248, 255). Apparent mineralocorticoid excess (AME) is an AR condition that leads to reduced enzymatic activity of 11βHSD2 due to loss-of-function mutations or epigenetic changes in the HSD11B2 gene (256-258). The deficient gene alters the inactivation of cortisol in the target renal cells leading to activation of MCR, which leads to renal Na retention, severe hypokalemia from kaliuresis and HTN. The severity of HTN correlates with the degree of loss of enzymatic activity (259, 260). Clinical presentation of AME may include growth retardation, short stature, HTN, and hypokalemia that can lead to diabetes insipidus (261). The typical presentation of AME includes childhood-onset HTN with hypokalemia, suppressed renin, very low to undetectable aldosterone levels (hyporeninemic hypoaldosteronism) and metabolic alkalosis. Heterozygotes usually develop HTN later in life, without the phenotypic characteristics of AME (262). The biochemical diagnosis can be made by profiling of urinary steroid metabolites, which shows decreased cortisol inactivation, with the urinary tetrahydrocortisol and tetrahydrocortisone ratio (THF + 5αTHF)/THE and nearly absent urinary free cortisone (259, 263). AME is responsive to low sodium diet and spironolactone therapy (259). Genetic testing for AME-associated loss-of-function mutations in HSD11B2 confirms the diagnosis. See Tables 9-11 for laboratory tests and ratios provided by questdiagnostics.com.
Liddle Syndrome (Pseudohyperaldosteronism)
Liddle syndrome is a rare AD form of early-onset monogenic HTN with a prevalence of 1.52% in young hypertensives (264). The condition is caused by gain-of-function mutations in the genes (16p13) encoding β (SCNN1B) and γ (SCNN1G) subunits of the epithelial sodium channel (ENaC) (265-267). ENac is rate limiting for Na absorption in the aldosterone-sensitive distal nephron comprising the late distal convoluted tubule, the connecting tubule, and the entire collecting duct (268, 269). As a consequence, increased renal Na reabsorption with subsequent volume expansion and kaliuresis leads to severe HTN, hypokalemia and metabolic alkalosis (267). The typical biochemical profile is ↓K, ↑urinary K, ↓PRA, and suppressed aldosterone levels (hyporeninemic hypoaldosteronism). HTN usually responds to a combination of salt restriction (<100 mmol/day) and amiloride or triamterene therapy. Hyporeninemic hypoaldosteronism may present in the elderly population and mimic the biochemical patterns of Liddle syndrome (270). However, true Liddle syndrome may be underappreciated and undiagnosed in adults (271). Genetic testing can identify the disease mutations (266, 267). Screening of Liddle syndrome should be encouraged in young hypertensives, particularly those with early penetrance, hypokalemia, and low renin levels after exclusion of common secondary causes (264). See Tables 9-11 for laboratory tests and ratios provided by questdiagnostics.com.
Pseudohypoaldosteronism Type 2
Pseudohypoaldosteronism type 2 (PHA-2), or Gordon syndrome, is an AD condition that is caused by loss-of-function mutations in WNK1 or WNK4, which are part of a family of serine-threonine protein kinases. Mutations in WNK1 or WNK4 lead to an increased activity of the NaCl cotransporter in the distal tubule and consequently Na and fluid retention. More recently, mutations in the KLHL3, CUL3, and SPAK genes have been linked to Gordon syndrome (272, 273). Clinical features of patients with this syndrome include short stature, hyperchloremic metabolic acidosis, normal aldosterone levels and severe HTN. Biochemical profile includes ↑↑K, hyperchloremic metabolic acidosis, normal or ↓aldosterone, ↓PRA, ↓serum HCO3 (variable in children), and hypercalciuria (occasionally). The condition is confirmed by sequencing of WNK1 or WNK4, or the other rare genes implicated. See Tables 9-11 for laboratory tests and ratios provided by questdiagnostics.com.
Pseudohypoaldosteronism Type 1
Pseudohypoaldosteronism type 1 (PHA-1) is a rare AR form of monogenic HTN that is characterized by resistance to aldosterone. In affected patients, aldosterone levels are normal or elevated, but the renal response to aldosterone is disrupted due to functional abnormalities in either MCR (autosomal dominant or sporadic PHA-1; NR3C2 mutations) or the amiloride-sensitive ENaC (autosomal recessive PHA-1; SCNN1B mutations) (274, 275). Clinically, PHA-1 is characterized by Na wasting, failure to thrive, hyperkalemia, hypovolemia and metabolic acidosis (276). The diagnosis of PHA-1 may be missed until adulthood (274). See Tables 9-11 for laboratory tests and ratios provided by questdiagnostics.com.
Constitutive Activation Of The Mineralocorticoid Receptor (Geller Syndrome)
Geller syndrome is an AD condition caused by gain-of-function mutations (4q31) in the gene encoding the MCR. One report described early-onset HTN due to a mutation in the MCR that was markedly exacerbated in pregnancy (277). The striking feature of this disorder is a severe exacerbation of HTN and hypokalemia during pregnancy due to the agonistic activity of progesterone and other mineralocorticoid antagonists on the MCR (278). The presence of HTN in males and non-pregnant females suggests that other functional mineralocorticoids are present (278). Biochemical profile includes ↑K, ↓aldosterone, and ↓PRA. See Tables 9-11 for laboratory tests and ratios provided by questdiagnostics.com.
OTHER POTENTIAL CAUSES (OR “BIOMARKERS”) OF ENDOCRINE HYPERTENSION
- Insulin resistance without obesity
- Obesity with and without insulin resistance
- Growth hormone deficiency
- Growth hormone excess
- Testosterone deficiency (279)
- Testosterone excess including polycystic ovarian syndrome
- Thyrotoxicosis
- Hypothyroidism
- Primary hyperparathyroidism
- Vitamin D deficiency (280)
COLLECTION OF SPECIMENS
Collection of specimens, special instructions and method used for laboratory tests commonly used in the diagnosis of endocrine hypertension (see Table 9 and 10)
Table 9. Tests/Code* | Method | Specimen | Adult reference range** |
Aldosterone 24-hour U 19552X
|
LC-MS/MS |
· 5 ml refrigerated U · min 0.8 ml |
· 2.3-21 µg/24-h |
Aldosterone, serum 17181X |
LC-MS/MS |
· Red-top tube · 1 mL refrigerated · min 0.25 mL |
· Standing 8-10 AM: ≤28 ng/dL · Supine 8-10 AM: 3-16 ng/dl
|
Aldosterone / Plasma Renin Activity Ratio (ARR) CPT code: 82088 CPTcode: 84244 |
LC-MS/MS |
· Lavender-top tube · 1.8 mL frozen EDTA plasma · min 0.8 ml |
· The most commonly adopted cutoff is >30 |
CAH panel 1: 11-β Hydroxylase deficiency) 15269X |
LC-MS/MS |
· No additives red top tube · 0.6ml refrigerated · min 0.3 ml |
· 11-Deoxycortisol/ Cortisol ratio >100 · Androstenedione ↑ · Testosterone ↑ |
CAH panel 3: Aldosterone synthase deficiency 15273X |
RIA LC-MS/MS |
· No additives red top tube · 1.8ml refrigerated · min 0.8 ml |
· 18-OH Corticosterone/Aldosterone ratio >40 |
CAH panel 4 (females): 17-α Hydroxylase deficiency/ 15274X |
LC-MS/MS |
· No additives red top tube · 1.2ml refrigerated · min 0.6 ml |
· Progesterone/17-OH Progesterone ratio >6 · Aldosterone ↓ · Corticosterone ↑ · Cortisol ↓ · Estradiol ↓ |
CAH panel 8 (males): 17-α Hydroxylase deficiency 15279X |
LC-MS/MS |
· No additives red top tube · 0.8ml refrigerated · min 0.4 ml |
· Progesterone/17-OH Progesterone ratio >6 · Aldosterone ↓ · Corticosterone ↑ · Cortisol ↓ · Testosterone ↓ |
Catecholamines fractioned 24-hour, urine 39627X |
HPLC |
· 10 ml room temp aliquot · Collect 25 ml U with · 6N HCl/min 4.5 ml |
· Epinephrine: 2-24 µg/24h · Norepinephrine: 15-100 µg/24h · Total N+E: 26-121 µg/24h · Dopamine: 58-480 µg/24h |
Catecholamines fractioned, plasma 314X |
HPLC |
· 4 ml sodium heparin · min 2.5 ml |
· Epinephrine: upright <95 pg/ml, supine <50 pg/ml · Norepinephrine: upright 217-1109 pg/ml, supine 112-658 pg/ml · Dopamine: upright<20 pg/ml, supine<10 pg/ml · Total N+E: upright 242-1125 pg/ml, supine 123-671 pg/ml |
Corticosterone 6547X |
LC-MS/MS |
· No additives red top tube · 1 ml refrigerated · min 0.25 mL |
· 8-10 AM 59-1293 ng/dL |
Cortisol free, 24-hour urine 11280X |
LC-MS/MS |
· 2 ml frozen aliquot of 24-hour urine · min 0.5 ml |
· 4-50 µg/24-hour |
Deoxycorticosterone (DOC) 6559X |
RIA |
· No additives red top tube 3 ml refrigerated S/ min 1.1 ml |
· Men: 3.5-11.5 ng/dL · Women follicular phase 1.5-8.5 ng/dL · luteal phase 3.5-13 ng/dL |
*Available from Quest Diagnostics (www.questdiagnostics.com)
** Reference range for adults; P-plasma; U-urine; S-serum, RIA- extraction chromatography, radioimmunoassay; LC-MS/MS-liquid chromatography, tandem mass spectrometry
Table 10. Tests | Special instructions* |
Aldosterone 24-hour urine
|
· Collect urine in 10 g of boric acid. Refrigerate during collection. · Record 24-h volume on vial and request form |
Aldosterone
|
· Draw upright blood half an hour after patient sits up; results vary with sodium excretion, electrolytic balance and posture (standing or recumbent)
|
Aldosterone/Plasma Renin Activity Ratio (ARR) |
· Do not refrigerate the specimen; refrigeration causes falsely-high PRA results. · Samples are collected in the morning after the patient has been out of bed for ≥ 2 hours and after sitting 5 -15 minutes. · Dietary salt intake should not be restricted, and potassium should be normalized if possible. · The patient can continue therapy with verapamil, hydralazine, prazosin hydrochloride, doxazosin mesylate, and terazosin hydrochloride during testing |
CAH panel 1: 11-β Hydroxylase deficiency CAH panel 3: Aldosterone synthase deficiency CAH panel 4 (females): 17-α Hydroxylase deficiency CAH panel 8 (males): 17-α Hydroxylase deficiency
|
· Early morning specimen preferred (age, sex and time of specimen collection need to be specified)
|
Catecholamines fractioned, 24-hour urine |
· Urine must be collected with 25 mL 6 N Hydrochloric Acid. · It is advisable for the patients to be off medications 3 days prior to the test and avoid coffee, alcohol, tea, tobacco and strenuous exercise |
Catecholamines fractioned, plasma |
· Collect in a pre-chilled vacutainer · Centrifuge in a refrigerated centrifuge within 30 minutes of collection · Separate plasma and freeze immediately · Avoid coffee, alcohol, tea, tobacco and strenuous exercise · Overnight fasting is necessary |
Cortisol free, 24-hour urine | · No preservatives preferred. However 25 mL 6 N hydrochloric acid or 10 g boric acid may be used |
11-Deoxycortisol | · Early morning specimen preferred (age, sex and time of specimen collection need to be specified) |
Metanephrines, Fractionated plasma |
· Patient must refrain from using acetaminophen for 48 hours before testing. · Patient must refrain from using caffeine, medications, and tobacco, and from drinking coffee, tea or alcoholic beverages, for at least 4 hours before testing. |
Plasma renin activity (PRA) | · Collect and transport at room temperature. Centrifuge and freeze the plasma immediately. |
Tetrahydroaldosterone 24-hour urine |
· Refrigerate during collection. · No use for preservatives |
*Available from Quest Diagnostics ( www.questdiagnostics.com)
Table 11. The laboratory testing protocols for rare causes of endocrine hypertension
Disease | Laboratory testing* | Genetic testing |
CAH: 17α-OH deficiency
|
· ↑DOC, ↓11-deoxycortisol, ↓↓ aldosterone, ↓PRA, ↓K, ↓plasma 17-OHP, ↓testosterone, · ↑urinary100*THDOC/(THE+THF+5αTHF) and(THA+THB+5αTHB)/(THE+THF+5αTF)
|
CYP17A1 |
CAH: 11ß-OH deficiency
|
· Hypokalemia (variable), ↓PRA, ↓↓ aldosterone, (the degree of hyporeninemia may vary widely), ↓cortisol, ↑ACTH, ↑11-deoxycortisol, ↑DOC, ↑ 19-nor-DOC · ↑↑urinary 100*THS/(THE+THF+5αTHF) and 100*THDOC/(THE+THF+5αTHF)
|
CYP11B1
|
Apparent mineralocorticoid excess |
· ↑ 24-hour urinary free cortisol/cortisone and ↑urinary (THF+5αTHF)/THE · check the level of tritiated water in plasma samples when 11-tritiated cortisol is injected
|
11ßHSD2 |
Liddle syndrome
|
· ↓ K, ↑urinary K, ↓PRA, suppressed aldosterone secretion, metabolic acidosis · ↓urinary THALDO (<2 µg/24h), normal steroid profile (24-hour urine cortisone/cortisol and other ratios)
|
SCNN1B and SCNN1G |
Pseudohypo aldosteronism Type 2 |
· ↑↑K, hyperchloremic metabolic acidosis, ↓aldosterone, ↓PRA, ↓serum HCO3 (variable in children), hypercalciuria (occasionally) · ↓urinary THALDO
|
WNK1 and WNK4
Rarely KLHL3, CUL3, and SPAK
|
Geller Syndrome
|
· ↑K, ↓aldosterone, ↓PRA · ↓urinary THALDO |
NR3C2 |
*Available from Quest Diagnostics ( www.questdiagnostics.com)
REFERENCES
- Nwankwo T, Yoon SS, Burt V, Gu Q. Hypertension among adults in the United States: National Health and Nutrition Examination Survey, 2011-2012. NCHS data brief. 2013(133):1-8.
- Fields LE, Burt VL, Cutler JA, Hughes J, Roccella EJ, Sorlie P. The burden of adult hypertension in the United States 1999 to 2000: a rising tide. Hypertension. 2004;44(4):398-404.
- Cutler JA, Sorlie PD, Wolz M, Thom T, Fields LE, Roccella EJ. Trends in hypertension prevalence, awareness, treatment, and control rates in United States adults between 1988-1994 and 1999-2004. Hypertension. 2008;52(5):818-27.
- Calhoun DA, Jones D, Textor S, Goff DC, Murphy TP, Toto RD, et al. Resistant hypertension: diagnosis, evaluation, and treatment. A scientific statement from the American Heart Association Professional Education Committee of the Council for High Blood Pressure Research. Hypertension. 2008;51(6):1403-19.
- Group SR, Wright JT, Jr., Williamson JD, Whelton PK, Snyder JK, Sink KM, et al. A Randomized Trial of Intensive versus Standard Blood-Pressure Control. N Engl J Med. 2015;373(22):2103-16.
- Yusuf S, Lonn E, Pais P, Bosch J, Lopez-Jaramillo P, Zhu J, et al. Blood-Pressure and Cholesterol Lowering in Persons without Cardiovascular Disease. N Engl J Med. 2016;374(21):2032-43.
- de Abreu-Silva EO, Beltrami-Moreira M. Sleep apnea: an underestimated cause of resistant hypertension. Current hypertension reviews. 2014;10(1):2-7.
- Goodfriend TL, Calhoun DA. Resistant hypertension, obesity, sleep apnea, and aldosterone: theory and therapy. Hypertension. 2004;43(3):518-24.
- James PA, Oparil S, Carter BL, Cushman WC, Dennison-Himmelfarb C, Handler J, et al. 2014 evidence-based guideline for the management of high blood pressure in adults: report from the panel members appointed to the Eighth Joint National Committee (JNC 8). JAMA. 2014;311(5):507-20.
- Reisin E, Harris RC, Rahman M. Commentary on the 2014 BP guidelines from the panel appointed to the Eighth Joint National Committee (JNC 8). Journal of the American Society of Nephrology : JASN. 2014;25(11):2419-24.
- Hypertension: The Clinical Management of Primary Hypertension in Adults: Update of Clinical Guidelines 18 and 34. National Institute for Health and Clinical Excellence: Guidance. London2011.
- Mancia G, Fagard R, Narkiewicz K, Redon J, Zanchetti A, Bohm M, et al. 2013 ESH/ESC Guidelines for the management of arterial hypertension: the Task Force for the management of arterial hypertension of the European Society of Hypertension (ESH) and of the European Society of Cardiology (ESC). J Hypertens. 2013;31(7):1281-357.
- Weber MA, Schiffrin EL, White WB, Mann S, Lindholm LH, Kenerson JG, et al. Clinical practice guidelines for the management of hypertension in the community: a statement by the American Society of Hypertension and the International Society of Hypertension. Journal of clinical hypertension. 2014;16(1):14-26.
- Daskalopoulou SS, Rabi DM, Zarnke KB, Dasgupta K, Nerenberg K, Cloutier L, et al. The 2015 Canadian Hypertension Education Program recommendations for blood pressure measurement, diagnosis, assessment of risk, prevention, and treatment of hypertension. The Canadian journal of cardiology. 2015;31(5):549-68.
- Stergiou GS, Ntineri A, Kollias A. Management of Masked Hypertension: Why Are We Still Sitting on the Fence? Hypertension. 2016.
- Burnier M. Resistant Hypertension: Is the Number of Drugs a Reliable Marker of Resistance? Hypertension. 2016.
- Calhoun DA, Jones D, Textor S, Goff DC, Murphy TP, Toto RD, et al. Resistant hypertension: diagnosis, evaluation, and treatment: a scientific statement from the American Heart Association Professional Education Committee of the Council for High Blood Pressure Research. Circulation. 2008;117(25):e510-26.
- Moser M, Setaro JF. Clinical practice. Resistant or difficult-to-control hypertension. N Engl J Med. 2006;355(4):385-92.
- Papadopoulos DP, Makris TK. Masked hypertension definition, impact, outcomes: a critical review. Journal of clinical hypertension. 2007;9(12):956-63.
- Bromfield SG, Shimbo D, Booth JN, 3rd, Correa A, Ogedegbe G, Carson AP, et al. Cardiovascular Risk Factors and Masked Hypertension: The Jackson Heart Study. Hypertension. 2016.
- Franklin SS, Thijs L, Hansen TW, O'Brien E, Staessen JA. White-coat hypertension: new insights from recent studies. Hypertension. 2013;62(6):982-7.
- Acelajado MC, Pisoni R, Dudenbostel T, Dell'Italia LJ, Cartmill F, Zhang B, et al. Refractory hypertension: definition, prevalence, and patient characteristics. Journal of clinical hypertension. 2012;14(1):7-12.
- Bavishi C, Goel S, Messerli FH. Isolated Systolic Hypertension: An Update After SPRINT. The American journal of medicine. 2016;129(12):1251-8.
- Funder JW, Carey RM, Mantero F, Murad MH, Reincke M, Shibata H, et al. The Management of Primary Aldosteronism: Case Detection, Diagnosis, and Treatment: An Endocrine Society Clinical Practice Guideline. J Clin Endocrinol Metab. 2016;101(5):1889-916.
- Funder JW. Primary Aldosteronism: Seismic Shifts. J Clin Endocrinol Metab. 2015;100(8):2853-5.
- Anderson GH, Jr., Blakeman N, Streeten DH. The effect of age on prevalence of secondary forms of hypertension in 4429 consecutively referred patients. J Hypertens. 1994;12(5):609-15.
- Hannah-Shmouni F, Stratakis CA, Koch CA. Flushing in (neuro)endocrinology. Reviews in endocrine & metabolic disorders. 2016.
- Turnbull JM. The rational clinical examination. Is listening for abdominal bruits useful in the evaluation of hypertension? JAMA. 1995;274(16):1299-301.
- Berglund G, Andersson O, Wilhelmsen L. Prevalence of primary and secondary hypertension: studies in a random population sample. British medical journal. 1976;2(6035):554-6.
- Piecha G, Wiecek A, Januszewicz A. Epidemiology and optimal management in patients with renal artery stenosis. Journal of nephrology. 2012;25(6):872-8.
- Safian RD, Textor SC. Renal-artery stenosis. N Engl J Med. 2001;344(6):431-42.
- Senitko M, Fenves AZ. An update on renovascular hypertension. Current cardiology reports. 2005;7(6):405-11.
- Krumme B, Donauer J. Atherosclerotic renal artery stenosis and reconstruction. Kidney international. 2006;70(9):1543-7.
- Gandhi SK, Powers JC, Nomeir AM, Fowle K, Kitzman DW, Rankin KM, et al. The pathogenesis of acute pulmonary edema associated with hypertension. N Engl J Med. 2001;344(1):17-22.
- Hirsch AT, Haskal ZJ, Hertzer NR, Bakal CW, Creager MA, Halperin JL, et al. ACC/AHA 2005 guidelines for the management of patients with peripheral arterial disease (lower extremity, renal, mesenteric, and abdominal aortic): executive summary a collaborative report from the American Association for Vascular Surgery/Society for Vascular Surgery, Society for Cardiovascular Angiography and Interventions, Society for Vascular Medicine and Biology, Society of Interventional Radiology, and the ACC/AHA Task Force on Practice Guidelines (Writing Committee to Develop Guidelines for the Management of Patients With Peripheral Arterial Disease) endorsed by the American Association of Cardiovascular and Pulmonary Rehabilitation; National Heart, Lung, and Blood Institute; Society for Vascular Nursing; TransAtlantic Inter-Society Consensus; and Vascular Disease Foundation. J Am Coll Cardiol. 2006;47(6):1239-312.
- Derkx FH, Schalekamp MA. Renal artery stenosis and hypertension. Lancet. 1994;344(8917):237-9.
- Petruzzelli M, Taylor KP, Koo B, Brown MJ. Telling Tails: Very High Plasma Renin Levels Prompt the Diagnosis of Renal Artery Stenosis, Despite Initial Negative Imaging. Hypertension. 2016;68(1):11-6.
- Brown MJ. Clinical value of plasma renin estimation in the management of hypertension. American journal of hypertension. 2014;27(8):1013-6.
- Vasbinder GB, Nelemans PJ, Kessels AG, Kroon AA, de Leeuw PW, van Engelshoven JM. Diagnostic tests for renal artery stenosis in patients suspected of having renovascular hypertension: a meta-analysis. Annals of internal medicine. 2001;135(6):401-11.
- Schreier DZ, Weaver FA, Frankhouse J, Papanicolaou G, Shore E, Yellin AE, et al. A prospective study of carbon dioxide-digital subtraction vs standard contrast arteriography in the evaluation of the renal arteries. Archives of surgery. 1996;131(5):503-7; discussion 7-8.
- Amis ES, Jr., Bigongiari LR, Bluth EI, Bush WH, Jr., Choyke PL, Fritzsche P, et al. Radiologic investigation of patients with renovascular hypertension. American College of Radiology. ACR Appropriateness Criteria. Radiology. 2000;215 Suppl:663-70.
- Beregi JP, Elkohen M, Deklunder G, Artaud D, Coullet JM, Wattinne L. Helical CT angiography compared with arteriography in the detection of renal artery stenosis. AJR American journal of roentgenology. 1996;167(2):495-501.
- Postma CT, van Aalen J, de Boo T, Rosenbusch G, Thien T. Doppler ultrasound scanning in the detection of renal artery stenosis in hypertensive patients. The British journal of radiology. 1992;65(778):857-60.
- Radermacher J, Chavan A, Schaffer J, Stoess B, Vitzthum A, Kliem V, et al. Detection of significant renal artery stenosis with color Doppler sonography: combining extrarenal and intrarenal approaches to minimize technical failure. Clinical nephrology. 2000;53(5):333-43.
- De Cobelli F, Venturini M, Vanzulli A, Sironi S, Salvioni M, Angeli E, et al. Renal arterial stenosis: prospective comparison of color Doppler US and breath-hold, three-dimensional, dynamic, gadolinium-enhanced MR angiography. Radiology. 2000;214(2):373-80.
- Mann SJ, Pickering TG, Sos TA, Uzzo RG, Sarkar S, Friend K, et al. Captopril renography in the diagnosis of renal artery stenosis: accuracy and limitations. The American journal of medicine. 1991;90(1):30-40.
- Olin JW, Piedmonte MR, Young JR, DeAnna S, Grubb M, Childs MB. The utility of duplex ultrasound scanning of the renal arteries for diagnosing significant renal artery stenosis. Annals of internal medicine. 1995;122(11):833-8.
- Broome DR, Girguis MS, Baron PW, Cottrell AC, Kjellin I, Kirk GA. Gadodiamide-associated nephrogenic systemic fibrosis: why radiologists should be concerned. AJR American journal of roentgenology. 2007;188(2):586-92.
- Sadowski EA, Bennett LK, Chan MR, Wentland AL, Garrett AL, Garrett RW, et al. Nephrogenic systemic fibrosis: risk factors and incidence estimation. Radiology. 2007;243(1):148-57.
- Setaro JF, Chen CC, Hoffer PB, Black HR. Captopril renography in the diagnosis of renal artery stenosis and the prediction of improvement with revascularization. The Yale Vascular Center experience. American journal of hypertension. 1991;4(12 Pt 2):698S-705S.
- Pedersen EB. Angiotensin-converting enzyme inhibitor renography. Pathophysiological, diagnostic and therapeutic aspects in renal artery stenosis. Nephrology, dialysis, transplantation : official publication of the European Dialysis and Transplant Association - European Renal Association. 1994;9(5):482-92.
- Etxabe J, Vazquez JA. Morbidity and mortality in Cushing's disease: an epidemiological approach. Clinical endocrinology. 1994;40(4):479-84.
- Lindholm J, Juul S, Jorgensen JO, Astrup J, Bjerre P, Feldt-Rasmussen U, et al. Incidence and late prognosis of cushing's syndrome: a population-based study. J Clin Endocrinol Metab. 2001;86(1):117-23.
- Singer J, Werner F, Koch CA, Bartels M, Aigner T, Lincke T, et al. Ectopic Cushing's syndrome caused by a well differentiated ACTH-secreting neuroendocrine carcinoma of the ileum. Experimental and clinical endocrinology & diabetes : official journal, German Society of Endocrinology [and] German Diabetes Association. 2010;118(8):524-9.
- Stratakis CA, Boikos SA. Genetics of adrenal tumors associated with Cushing's syndrome: a new classification for bilateral adrenocortical hyperplasias. Nature clinical practice Endocrinology & metabolism. 2007;3(11):748-57.
- Lodish M, Stratakis CA. A genetic and molecular update on adrenocortical causes of Cushing syndrome. Nature reviews Endocrinology. 2016;12(5):255-62.
- Kirschner MA, Powell RD, Jr., Lipsett MB. Cushing's Syndrome: Nodular Cortical Hyperplasia of Adrenal Glands with Clinical and Pathological Features Suggesting Adrenocortical Tumor. J Clin Endocrinol Metab. 1964;24:947-55.
- Fragoso MC, Alencar GA, Lerario AM, Bourdeau I, Almeida MQ, Mendonca BB, et al. Genetics of primary macronodular adrenal hyperplasia. The Journal of endocrinology. 2015;224(1):R31-43.
- Alencar GA, Lerario AM, Nishi MY, Mariani BM, Almeida MQ, Tremblay J, et al. ARMC5 mutations are a frequent cause of primary macronodular adrenal Hyperplasia. J Clin Endocrinol Metab. 2014;99(8):E1501-9.
- Assie G, Libe R, Espiard S, Rizk-Rabin M, Guimier A, Luscap W, et al. ARMC5 mutations in macronodular adrenal hyperplasia with Cushing's syndrome. N Engl J Med. 2013;369(22):2105-14.
- Faucz FR, Zilbermint M, Lodish MB, Szarek E, Trivellin G, Sinaii N, et al. Macronodular adrenal hyperplasia due to mutations in an armadillo repeat containing 5 (ARMC5) gene: a clinical and genetic investigation. J Clin Endocrinol Metab. 2014;99(6):E1113-9.
- Stratakis CA. Diagnosis and Clinical Genetics of Cushing Syndrome in Pediatrics. Endocrinology and metabolism clinics of North America. 2016;45(2):311-28.
- Lacroix A, Feelders RA, Stratakis CA, Nieman LK. Cushing's syndrome. Lancet. 2015;386(9996):913-27.
- Nieman LK, Biller BM, Findling JW, Murad MH, Newell-Price J, Savage MO, et al. Treatment of Cushing's Syndrome: An Endocrine Society Clinical Practice Guideline. J Clin Endocrinol Metab. 2015;100(8):2807-31.
- Nieman LK, Biller BM, Findling JW, Newell-Price J, Savage MO, Stewart PM, et al. The diagnosis of Cushing's syndrome: an Endocrine Society Clinical Practice Guideline. J Clin Endocrinol Metab. 2008;93(5):1526-40.
- Afshari A, Ardeshirpour Y, Lodish MB, Gourgari E, Sinaii N, Keil M, et al. Facial Plethora: Modern Technology for Quantifying an Ancient Clinical Sign and Its Use in Cushing Syndrome. J Clin Endocrinol Metab. 2015;100(10):3928-33.
- El Ghorayeb N, Bourdeau I, Lacroix A. Multiple aberrant hormone receptors in Cushing's syndrome. Eur J Endocrinol. 2015;173(4):M45-60.
- Glass AR, Zavadil AP, 3rd, Halberg F, Cornelissen G, Schaaf M. Circadian rhythm of serum cortisol in Cushing's disease. J Clin Endocrinol Metab. 1984;59(1):161-5.
- Refetoff S, Van Cauter E, Fang VS, Laderman C, Graybeal ML, Landau RL. The effect of dexamethasone on the 24-hour profiles of adrenocorticotropin and cortisol in Cushing's syndrome. J Clin Endocrinol Metab. 1985;60(3):527-35.
- Liu H, Bravata DM, Cabaccan J, Raff H, Ryzen E. Elevated late-night salivary cortisol levels in elderly male type 2 diabetic veterans. Clinical endocrinology. 2005;63(6):642-9.
- Meikle AW. Dexamethasone suppression tests: usefulness of simultaneous measurement of plasma cortisol and dexamethasone. Clinical endocrinology. 1982;16(4):401-8.
- Qureshi AC, Bahri A, Breen LA, Barnes SC, Powrie JK, Thomas SM, et al. The influence of the route of oestrogen administration on serum levels of cortisol-binding globulin and total cortisol. Clinical endocrinology. 2007;66(5):632-5.
- Wood PJ, Barth JH, Freedman DB, Perry L, Sheridan B. Evidence for the low dose dexamethasone suppression test to screen for Cushing's syndrome--recommendations for a protocol for biochemistry laboratories. Annals of clinical biochemistry. 1997;34 ( Pt 3):222-9.
- Pecori Giraldi F, Ambrogio AG, De Martin M, Fatti LM, Scacchi M, Cavagnini F. Specificity of first-line tests for the diagnosis of Cushing's syndrome: assessment in a large series. J Clin Endocrinol Metab. 2007;92(11):4123-9.
- Fassnacht M, Arlt W, Bancos I, Dralle H, Newell-Price J, Sahdev A, et al. Management of adrenal incidentalomas: European Society of Endocrinology Clinical Practice Guideline in collaboration with the European Network for the Study of Adrenal Tumors. Eur J Endocrinol. 2016;175(2):G1-G34.
- Lin CL, Wu TJ, Machacek DA, Jiang NS, Kao PC. Urinary free cortisol and cortisone determined by high performance liquid chromatography in the diagnosis of Cushing's syndrome. J Clin Endocrinol Metab. 1997;82(1):151-5.
- Nieman LK, Oldfield EH, Wesley R, Chrousos GP, Loriaux DL, Cutler GB, Jr. A simplified morning ovine corticotropin-releasing hormone stimulation test for the differential diagnosis of adrenocorticotropin-dependent Cushing's syndrome. J Clin Endocrinol Metab. 1993;77(5):1308-12.
- Sheldon WR, Jr., DeBold CR, Evans WS, DeCherney GS, Jackson RV, Island DP, et al. Rapid sequential intravenous administration of four hypothalamic releasing hormones as a combined anterior pituitary function test in normal subjects. J Clin Endocrinol Metab. 1985;60(4):623-30.
- Landolt AM, Schubiger O, Maurer R, Girard J. The value of inferior petrosal sinus sampling in diagnosis and treatment of Cushing's disease. Clinical endocrinology. 1994;40(4):485-92.
- Findling JW, Kehoe ME, Shaker JL, Raff H. Routine inferior petrosal sinus sampling in the differential diagnosis of adrenocorticotropin (ACTH)-dependent Cushing's syndrome: early recognition of the occult ectopic ACTH syndrome. J Clin Endocrinol Metab. 1991;73(2):408-13.
- Oldfield EH, Doppman JL, Nieman LK, Chrousos GP, Miller DL, Katz DA, et al. Petrosal sinus sampling with and without corticotropin-releasing hormone for the differential diagnosis of Cushing's syndrome. N Engl J Med. 1991;325(13):897-905.
- Kaltsas GA, Giannulis MG, Newell-Price JD, Dacie JE, Thakkar C, Afshar F, et al. A critical analysis of the value of simultaneous inferior petrosal sinus sampling in Cushing's disease and the occult ectopic adrenocorticotropin syndrome. J Clin Endocrinol Metab. 1999;84(2):487-92.
- Sharma ST, Nieman LK. Is prolactin measurement of value during inferior petrosal sinus sampling in patients with adrenocorticotropic hormone-dependent Cushing's Syndrome? J Endocrinol Invest. 2013;36(11):1112-6.
- Invitti C, Pecori Giraldi F, de Martin M, Cavagnini F. Diagnosis and management of Cushing's syndrome: results of an Italian multicentre study. Study Group of the Italian Society of Endocrinology on the Pathophysiology of the Hypothalamic-Pituitary-Adrenal Axis. J Clin Endocrinol Metab. 1999;84(2):440-8.
- Doppman JL, Frank JA, Dwyer AJ, Oldfield EH, Miller DL, Nieman LK, et al. Gadolinium DTPA enhanced MR imaging of ACTH-secreting microadenomas of the pituitary gland. Journal of computer assisted tomography. 1988;12(5):728-35.
- Patronas N, Bulakbasi N, Stratakis CA, Lafferty A, Oldfield EH, Doppman J, et al. Spoiled gradient recalled acquisition in the steady state technique is superior to conventional postcontrast spin echo technique for magnetic resonance imaging detection of adrenocorticotropin-secreting pituitary tumors. J Clin Endocrinol Metab. 2003;88(4):1565-9.
- Hall WA, Luciano MG, Doppman JL, Patronas NJ, Oldfield EH. Pituitary magnetic resonance imaging in normal human volunteers: occult adenomas in the general population. Annals of internal medicine. 1994;120(10):817-20.
- Escourolle H, Abecassis JP, Bertagna X, Guilhaume B, Pariente D, Derome P, et al. Comparison of computerized tomography and magnetic resonance imaging for the examination of the pituitary gland in patients with Cushing's disease. Clinical endocrinology. 1993;39(3):307-13.
- Isidori AM, Sbardella E, Zatelli MC, Boschetti M, Vitale G, Colao A, et al. Conventional and Nuclear Medicine Imaging in Ectopic Cushing's Syndrome: A Systematic Review. J Clin Endocrinol Metab. 2015;100(9):3231-44.
- Patel D, Gara SK, Ellis RJ, Boufraqech M, Nilubol N, Millo C, et al. FDG PET/CT Scan and Functional Adrenal Tumors: A Pilot Study for Lateralization. World journal of surgery. 2016;40(3):683-9.
- Boscaro M, Arnaldi G. Approach to the patient with possible Cushing's syndrome. J Clin Endocrinol Metab. 2009;94(9):3121-31.
- McKenzie TJ, Lillegard JB, Young WF, Jr., Thompson GB. Aldosteronomas--state of the art. The Surgical clinics of North America. 2009;89(5):1241-53.
- Conn JW, Cohen EL, Rovner DR. Landmark article Oct 19, 1964: Suppression of plasma renin activity in primary aldosteronism. Distinguishing primary from secondary aldosteronism in hypertensive disease. By Jerome W. Conn, Edwin L. Cohen and David R. Rovner. JAMA. 1985;253(4):558-66.
- Funder JW. Primary aldosteronism as a public health issue. The lancet Diabetes & endocrinology. 2016;4(12):972-3.
- Hannemann A, Bidlingmaier M, Friedrich N, Manolopoulou J, Spyroglou A, Volzke H, et al. Screening for primary aldosteronism in hypertensive subjects: results from two German epidemiological studies. Eur J Endocrinol. 2012;167(1):7-15.
- Schwartz GL, Turner ST. Screening for primary aldosteronism in essential hypertension: diagnostic accuracy of the ratio of plasma aldosterone concentration to plasma renin activity. Clinical chemistry. 2005;51(2):386-94.
- Mulatero P, Stowasser M, Loh KC, Fardella CE, Gordon RD, Mosso L, et al. Increased diagnosis of primary aldosteronism, including surgically correctable forms, in centers from five continents. J Clin Endocrinol Metab. 2004;89(3):1045-50.
- Rossi E, Regolisti G, Negro A, Sani C, Davoli S, Perazzoli F. High prevalence of primary aldosteronism using postcaptopril plasma aldosterone to renin ratio as a screening test among Italian hypertensives. American journal of hypertension. 2002;15(10 Pt 1):896-902.
- Funder JW. Genetic disorders in primary aldosteronism-familial and somatic. The Journal of steroid biochemistry and molecular biology. 2017;165(Pt A):154-7.
- Gordon RD, Tunny TJ. Aldosterone-producing-adenoma (A-P-A): effect of pregnancy. Clinical and experimental hypertension Part A, Theory and practice. 1982;4(9-10):1685-93.
- Stowasser M, Gordon RD. Primary aldosteronism--careful investigation is essential and rewarding. Molecular and cellular endocrinology. 2004;217(1-2):33-9.
- Williams JS, Williams GH, Raji A, Jeunemaitre X, Brown NJ, Hopkins PN, et al. Prevalence of primary hyperaldosteronism in mild to moderate hypertension without hypokalaemia. Journal of human hypertension. 2006;20(2):129-36.
- Baudrand R, Guarda FJ, Torrey J, Williams G, Vaidya A. Dietary Sodium Restriction Increases the Risk of Misinterpreting Mild Cases of Primary Aldosteronism. J Clin Endocrinol Metab. 2016;101(11):3989-96.
- Mulatero P, Rabbia F, Milan A, Paglieri C, Morello F, Chiandussi L, et al. Drug effects on aldosterone/plasma renin activity ratio in primary aldosteronism. Hypertension. 2002;40(6):897-902.
- Buhler FR, Laragh JH, Baer L, Vaughan ED, Jr., Brunner HR. Propranolol inhibition of renin secretion. A specific approach to diagnosis and treatment of renin-dependent hypertensive diseases. N Engl J Med. 1972;287(24):1209-14.
- Gordon MS, Williams GH, Hollenberg NK. Renal and adrenal responsiveness to angiotensin II: influence of beta adrenergic blockade. Endocrine research. 1992;18(2):115-31.
- Cappuccio FP, Markandu ND, Sagnella GA, Singer DR, Buckley MG, Miller MA, et al. Effects of amlodipine on urinary sodium excretion, renin-angiotensin-aldosterone system, atrial natriuretic peptide and blood pressure in essential hypertension. Journal of human hypertension. 1991;5(2):115-9.
- Brown MJ, Hopper RV. Calcium-channel blockade can mask the diagnosis of Conn's syndrome. Postgraduate medical journal. 1999;75(882):235-6.
- Mantero F, Fallo F, Opocher G, Armanini D, Boscaro M, Scaroni C. Effect of angiotensin II and converting enzyme inhibitor (captopril) on blood pressure, plasma renin activity and aldosterone in primary aldosteronism. Clinical science. 1981;61 Suppl 7:289s-93s.
- Baudrand R, Pojoga LH, Vaidya A, Garza AE, Vohringer PA, Jeunemaitre X, et al. Statin Use and Adrenal Aldosterone Production in Hypertensive and Diabetic Subjects. Circulation. 2015;132(19):1825-33.
- Gallay BJ, Ahmad S, Xu L, Toivola B, Davidson RC. Screening for primary aldosteronism without discontinuing hypertensive medications: plasma aldosterone-renin ratio. American journal of kidney diseases : the official journal of the National Kidney Foundation. 2001;37(4):699-705.
- Campbell DJ, Nussberger J, Stowasser M, Danser AH, Morganti A, Frandsen E, et al. Activity assays and immunoassays for plasma Renin and prorenin: information provided and precautions necessary for accurate measurement. Clinical chemistry. 2009;55(5):867-77.
- Racine MC, Douville P, Lebel M. Functional tests for primary aldosteronism: value of captopril suppression. Curr Hypertens Rep. 2002;4(3):245-9.
- Castro OL, Yu X, Kem DC. Diagnostic value of the post-captopril test in primary aldosteronism. Hypertension. 2002;39(4):935-8.
- Tsiavos V, Markou A, Papanastasiou L, Kounadi T, Androulakis, II, Voulgaris N, et al. A new highly sensitive and specific overnight combined screening and diagnostic test for primary aldosteronism. Eur J Endocrinol. 2016;175(1):21-8.
- Brown JM, Williams JS, Luther JM, Garg R, Garza AE, Pojoga LH, et al. Human interventions to characterize novel relationships between the renin-angiotensin-aldosterone system and parathyroid hormone. Hypertension. 2014;63(2):273-80.
- Brown J, de Boer IH, Robinson-Cohen C, Siscovick DS, Kestenbaum B, Allison M, et al. Aldosterone, parathyroid hormone, and the use of renin-angiotensin-aldosterone system inhibitors: the multi-ethnic study of atherosclerosis. J Clin Endocrinol Metab. 2015;100(2):490-9.
- Agharazii M, Douville P, Grose JH, Lebel M. Captopril suppression versus salt loading in confirming primary aldosteronism. Hypertension. 2001;37(6):1440-3.
- Holland OB, Brown H, Kuhnert L, Fairchild C, Risk M, Gomez-Sanchez CE. Further evaluation of saline infusion for the diagnosis of primary aldosteronism. Hypertension. 1984;6(5):717-23.
- Litchfield WR, Dluhy RG. Primary aldosteronism. Endocrinology and metabolism clinics of North America. 1995;24(3):593-612.
- Rossi GP, Belfiore A, Bernini G, Desideri G, Fabris B, Ferri C, et al. Comparison of the captopril and the saline infusion test for excluding aldosterone-producing adenoma. Hypertension. 2007;50(2):424-31.
- Wolley MJ, Ahmed AH, Gordon RD, Stowasser M. Does ACTH improve the diagnostic performance of adrenal vein sampling for subtyping primary aldosteronism? Clinical endocrinology. 2016;85(5):703-9.
- Shibayama Y, Wada N, Umakoshi H, Ichijo T, Fujii Y, Kamemura K, et al. Bilateral aldosterone suppression and its resolution in adrenal vein sampling of patients with primary aldosteronism: analysis of data from the WAVES-J study. Clinical endocrinology. 2016;85(5):696-702.
- Goupil R, Wolley M, Ungerer J, McWhinney B, Mukai K, Naruse M, et al. Use of plasma metanephrine to aid adrenal venous sampling in combined aldosterone and cortisol over-secretion. Endocrinology, diabetes & metabolism case reports. 2015;2015:150075.
- Freel EM, Stanson AW, Thompson GB, Grant CS, Farley DR, Richards ML, et al. Adrenal venous sampling for catecholamines: a normal value study. J Clin Endocrinol Metab. 2010;95(3):1328-32.
- Mailhot JP, Traistaru M, Soulez G, Ladouceur M, Giroux MF, Gilbert P, et al. Adrenal Vein Sampling in Primary Aldosteronism: Sensitivity and Specificity of Basal Adrenal Vein to Peripheral Vein Cortisol and Aldosterone Ratios to Confirm Catheterization of the Adrenal Vein. Radiology. 2015;277(3):887-94.
- Rossi GP, Sacchetto A, Chiesura-Corona M, De Toni R, Gallina M, Feltrin GP, et al. Identification of the etiology of primary aldosteronism with adrenal vein sampling in patients with equivocal computed tomography and magnetic resonance findings: results in 104 consecutive cases. J Clin Endocrinol Metab. 2001;86(3):1083-90.
- Wolley M, Ahmed A, Gordon R, Stowasser M. 9b.04: Does Contralateral Suppression at Adrenal Venous Sampling Predict Outcome Following Unilateral Adrenalectomy for Primary Aldosteronism? A Retrospective Study. J Hypertens. 2015;33 Suppl 1:e121.
- Umakoshi H, Tanase-Nakao K, Wada N, Ichijo T, Sone M, Inagaki N, et al. Importance of contralateral aldosterone suppression during adrenal vein sampling in the subtype evaluation of primary aldosteronism. Clinical endocrinology. 2015;83(4):462-7.
- El Ghorayeb N, Mazzuco TL, Bourdeau I, Mailhot JP, Zhu PS, Therasse E, et al. Basal and Post-ACTH Aldosterone and Its Ratios Are Useful During Adrenal Vein Sampling in Primary Aldosteronism. J Clin Endocrinol Metab. 2016;101(4):1826-35.
- Durivage C, Blanchette R, Soulez G, Chagnon M, Gilbert P, Giroux MF, et al. Adrenal venous sampling in primary aldosteronism: multinomial regression modeling to detect aldosterone secretion lateralization when right adrenal sampling is missing. J Hypertens. 2016.
- Eisenhofer G, Dekkers T, Peitzsch M, Dietz AS, Bidlingmaier M, Treitl M, et al. Mass Spectrometry-Based Adrenal and Peripheral Venous Steroid Profiling for Subtyping Primary Aldosteronism. Clinical chemistry. 2016;62(3):514-24.
- Sukor N, Gordon RD, Ku YK, Jones M, Stowasser M. Role of unilateral adrenalectomy in bilateral primary aldosteronism: a 22-year single center experience. J Clin Endocrinol Metab. 2009;94(7):2437-45.
- Ahmed AH, Gordon RD, Sukor N, Pimenta E, Stowasser M. Quality of life in patients with bilateral primary aldosteronism before and during treatment with spironolactone and/or amiloride, including a comparison with our previously published results in those with unilateral disease treated surgically. J Clin Endocrinol Metab. 2011;96(9):2904-11.
- Zarnegar R, Young WF, Jr., Lee J, Sweet MP, Kebebew E, Farley DR, et al. The aldosteronoma resolution score: predicting complete resolution of hypertension after adrenalectomy for aldosteronoma. Annals of surgery. 2008;247(3):511-8.
- Rossi GP, Auchus RJ, Brown M, Lenders JW, Naruse M, Plouin PF, et al. An expert consensus statement on use of adrenal vein sampling for the subtyping of primary aldosteronism. Hypertension. 2014;63(1):151-60.
- Dekkers T, Prejbisz A, Kool LJ, Groenewoud HJ, Velema M, Spiering W, et al. Adrenal vein sampling versus CT scan to determine treatment in primary aldosteronism: an outcome-based randomised diagnostic trial. The lancet Diabetes & endocrinology. 2016;4(9):739-46.
- Suzuki K, Fujita K, Ushiyama T, Mugiya S, Kageyama S, Ishikawa A. Efficacy of an ultrasonic surgical system for laparoscopic adrenalectomy. The Journal of urology. 1995;154(2 Pt 1):484-6.
- Abrams HL, Siegelman SS, Adams DF, Sanders R, Finberg HJ, Hessel SJ, et al. Computed tomography versus ultrasound of the adrenal gland: a prospective study. Radiology. 1982;143(1):121-8.
- Suzuki Y, Sasagawa, Suzuki H, Izumi T, Kaneko H, Nakada T. The role of ultrasonography in the detection of adrenal masses: comparison with computed tomography and magnetic resonance imaging. International urology and nephrology. 2001;32(3):303-6.
- Fontana D, Porpiglia F, Destefanis P, Fiori C, Ali A, Terzolo M, et al. What is the role of ultrasonography in the follow-up of adrenal incidentalomas? The Gruppo Piemontese Incidentalomi Surrenalici. Urology. 1999;54(4):612-6.
- Rubello D, Bui C, Casara D, Gross MD, Fig LM, Shapiro B. Functional scintigraphy of the adrenal gland. Eur J Endocrinol. 2002;147(1):13-28.
- Falke TH, Sandler MP. Classification of silent adrenal masses: time to get practical. Journal of nuclear medicine : official publication, Society of Nuclear Medicine. 1994;35(7):1152-4.
- Gross MD, Shapiro B, Bouffard JA, Glazer GM, Francis IR, Wilton GP, et al. Distinguishing benign from malignant euadrenal masses. Annals of internal medicine. 1988;109(8):613-8.
- Gross MD, Shapiro B, Francis IR, Glazer GM, Bree RL, Arcomano MA, et al. Scintigraphic evaluation of clinically silent adrenal masses. Journal of nuclear medicine : official publication, Society of Nuclear Medicine. 1994;35(7):1145-52.
- Daunt N. Adrenal vein sampling: how to make it quick, easy, and successful. Radiographics : a review publication of the Radiological Society of North America, Inc. 2005;25 Suppl 1:S143-58.
- Blake MA, Kalra MK, Sweeney AT, Lucey BC, Maher MM, Sahani DV, et al. Distinguishing benign from malignant adrenal masses: multi-detector row CT protocol with 10-minute delay. Radiology. 2006;238(2):578-85.
- Young WF, Jr. Conventional imaging in adrenocortical carcinoma: update and perspectives. Hormones & cancer. 2011;2(6):341-7.
- McNicholas MM, Lee MJ, Mayo-Smith WW, Hahn PF, Boland GW, Mueller PR. An imaging algorithm for the differential diagnosis of adrenal adenomas and metastases. AJR American journal of roentgenology. 1995;165(6):1453-9.
- Mantero F, Terzolo M, Arnaldi G, Osella G, Masini AM, Ali A, et al. A survey on adrenal incidentaloma in Italy. Study Group on Adrenal Tumors of the Italian Society of Endocrinology. J Clin Endocrinol Metab. 2000;85(2):637-44.
- Hamrahian AH, Ioachimescu AG, Remer EM, Motta-Ramirez G, Bogabathina H, Levin HS, et al. Clinical utility of noncontrast computed tomography attenuation value (hounsfield units) to differentiate adrenal adenomas/hyperplasias from nonadenomas: Cleveland Clinic experience. J Clin Endocrinol Metab. 2005;90(2):871-7.
- Lee MJ, Hahn PF, Papanicolaou N, Egglin TK, Saini S, Mueller PR, et al. Benign and malignant adrenal masses: CT distinction with attenuation coefficients, size, and observer analysis. Radiology. 1991;179(2):415-8.
- Korobkin M, Brodeur FJ, Francis IR, Quint LE, Dunnick NR, Londy F. CT time-attenuation washout curves of adrenal adenomas and nonadenomas. AJR American journal of roentgenology. 1998;170(3):747-52.
- Caoili EM, Korobkin M, Francis IR, Cohan RH, Platt JF, Dunnick NR, et al. Adrenal masses: characterization with combined unenhanced and delayed enhanced CT. Radiology. 2002;222(3):629-33.
- Szolar DH, Kammerhuber FH. Adrenal adenomas and nonadenomas: assessment of washout at delayed contrast-enhanced CT. Radiology. 1998;207(2):369-75.
- Kempers MJ, Lenders JW, van Outheusden L, van der Wilt GJ, Schultze Kool LJ, Hermus AR, et al. Systematic review: diagnostic procedures to differentiate unilateral from bilateral adrenal abnormality in primary aldosteronism. Annals of internal medicine. 2009;151(5):329-37.
- Pena CS, Boland GW, Hahn PF, Lee MJ, Mueller PR. Characterization of indeterminate (lipid-poor) adrenal masses: use of washout characteristics at contrast-enhanced CT. Radiology. 2000;217(3):798-802.
- Korobkin M, Lombardi TJ, Aisen AM, Francis IR, Quint LE, Dunnick NR, et al. Characterization of adrenal masses with chemical shift and gadolinium-enhanced MR imaging. Radiology. 1995;197(2):411-8.
- Outwater EK, Siegelman ES, Radecki PD, Piccoli CW, Mitchell DG. Distinction between benign and malignant adrenal masses: value of T1-weighted chemical-shift MR imaging. AJR American journal of roentgenology. 1995;165(3):579-83.
- Bilbey JH, McLoughlin RF, Kurkjian PS, Wilkins GE, Chan NH, Schmidt N, et al. MR imaging of adrenal masses: value of chemical-shift imaging for distinguishing adenomas from other tumors. AJR American journal of roentgenology. 1995;164(3):637-42.
- Heinz-Peer G, Honigschnabl S, Schneider B, Niederle B, Kaserer K, Lechner G. Characterization of adrenal masses using MR imaging with histopathologic correlation. AJR American journal of roentgenology. 1999;173(1):15-22.
- Lim V, Guo Q, Grant CS, Thompson GB, Richards ML, Farley DR, et al. Accuracy of adrenal imaging and adrenal venous sampling in predicting surgical cure of primary aldosteronism. J Clin Endocrinol Metab. 2014;99(8):2712-9.
- Boland GW, Goldberg MA, Lee MJ, Mayo-Smith WW, Dixon J, McNicholas MM, et al. Indeterminate adrenal mass in patients with cancer: evaluation at PET with 2-[F-18]-fluoro-2-deoxy-D-glucose. Radiology. 1995;194(1):131-4.
- Erasmus JJ, Patz EF, Jr., McAdams HP, Murray JG, Herndon J, Coleman RE, et al. Evaluation of adrenal masses in patients with bronchogenic carcinoma using 18F-fluorodeoxyglucose positron emission tomography. AJR American journal of roentgenology. 1997;168(5):1357-60.
- Maurea S, Mainolfi C, Bazzicalupo L, Panico MR, Imparato C, Alfano B, et al. Imaging of adrenal tumors using FDG PET: comparison of benign and malignant lesions. AJR American journal of roentgenology. 1999;173(1):25-9.
- Yun M, Kim W, Alnafisi N, Lacorte L, Jang S, Alavi A. 18F-FDG PET in characterizing adrenal lesions detected on CT or MRI. Journal of nuclear medicine : official publication, Society of Nuclear Medicine. 2001;42(12):1795-9.
- Tenenbaum F, Groussin L, Foehrenbach H, Tissier F, Gouya H, Bertherat J, et al. 18F-fluorodeoxyglucose positron emission tomography as a diagnostic tool for malignancy of adrenocortical tumours? Preliminary results in 13 consecutive patients. Eur J Endocrinol. 2004;150(6):789-92.
- Blake MA, Slattery JM, Kalra MK, Halpern EF, Fischman AJ, Mueller PR, et al. Adrenal lesions: characterization with fused PET/CT image in patients with proved or suspected malignancy--initial experience. Radiology. 2006;238(3):970-7.
- Minn H, Salonen A, Friberg J, Roivainen A, Viljanen T, Langsjo J, et al. Imaging of adrenal incidentalomas with PET using (11)C-metomidate and (18)F-FDG. Journal of nuclear medicine : official publication, Society of Nuclear Medicine. 2004;45(6):972-9.
- Hennings J, Lindhe O, Bergstrom M, Langstrom B, Sundin A, Hellman P. [11C]metomidate positron emission tomography of adrenocortical tumors in correlation with histopathological findings. J Clin Endocrinol Metab. 2006;91(4):1410-4.
- Burton TJ, Mackenzie IS, Balan K, Koo B, Bird N, Soloviev DV, et al. Evaluation of the sensitivity and specificity of (11)C-metomidate positron emission tomography (PET)-CT for lateralizing aldosterone secretion by Conn's adenomas. J Clin Endocrinol Metab. 2012;97(1):100-9.
- Powlson AS, Gurnell M, Brown MJ. Nuclear imaging in the diagnosis of primary aldosteronism. Current opinion in endocrinology, diabetes, and obesity. 2015;22(3):150-6.
- Abe T, Naruse M, Young WF, Jr., Kobashi N, Doi Y, Izawa A, et al. A Novel CYP11B2-Specific Imaging Agent for Detection of Unilateral Subtypes of Primary Aldosteronism. J Clin Endocrinol Metab. 2016;101(3):1008-15.
- Lifton RP, Dluhy RG, Powers M, Rich GM, Cook S, Ulick S, et al. A chimaeric 11 beta-hydroxylase/aldosterone synthase gene causes glucocorticoid-remediable aldosteronism and human hypertension. Nature. 1992;355(6357):262-5.
- O'Mahony S, Burns A, Murnaghan DJ. Dexamethasone-suppressible hyperaldosteronism: a large new kindred. Journal of human hypertension. 1989;3(4):255-8.
- Dluhy RG, Anderson B, Harlin B, Ingelfinger J, Lifton R. Glucocorticoid-remediable aldosteronism is associated with severe hypertension in early childhood. The Journal of pediatrics. 2001;138(5):715-20.
- Stowasser M, Bachmann AW, Huggard PR, Rossetti TR, Gordon RD. Severity of hypertension in familial hyperaldosteronism type I: relationship to gender and degree of biochemical disturbance. J Clin Endocrinol Metab. 2000;85(6):2160-6.
- Jeunemaitre X, Charru A, Pascoe L, Guyene TT, Aupetit-Faisant B, Shackleton CH, et al. [Hyperaldosteronism sensitive to dexamethasone with adrenal adenoma. Clinical, biological and genetic study]. Presse medicale. 1995;24(27):1243-8.
- Litchfield WR, Anderson BF, Weiss RJ, Lifton RP, Dluhy RG. Intracranial aneurysm and hemorrhagic stroke in glucocorticoid-remediable aldosteronism. Hypertension. 1998;31(1 Pt 2):445-50.
- Torpy DJ, Gordon RD, Lin JP, Huggard PR, Taymans SE, Stowasser M, et al. Familial hyperaldosteronism type II: description of a large kindred and exclusion of the aldosterone synthase (CYP11B2) gene. J Clin Endocrinol Metab. 1998;83(9):3214-8.
- Carss KJ, Stowasser M, Gordon RD, O'Shaughnessy KM. Further study of chromosome 7p22 to identify the molecular basis of familial hyperaldosteronism type II. Journal of human hypertension. 2011;25(9):560-4.
- Sukor N, Mulatero P, Gordon RD, So A, Duffy D, Bertello C, et al. Further evidence for linkage of familial hyperaldosteronism type II at chromosome 7p22 in Italian as well as Australian and South American families. J Hypertens. 2008;26(8):1577-82.
- Jeske YW, So A, Kelemen L, Sukor N, Willys C, Bulmer B, et al. Examination of chromosome 7p22 candidate genes RBaK, PMS2 and GNA12 in familial hyperaldosteronism type II. Clinical and experimental pharmacology & physiology. 2008;35(4):380-5.
- So A, Duffy DL, Gordon RD, Jeske YW, Lin-Su K, New MI, et al. Familial hyperaldosteronism type II is linked to the chromosome 7p22 region but also shows predicted heterogeneity. J Hypertens. 2005;23(8):1477-84.
- Geller DS, Zhang J, Wisgerhof MV, Shackleton C, Kashgarian M, Lifton RP. A novel form of human mendelian hypertension featuring nonglucocorticoid-remediable aldosteronism. J Clin Endocrinol Metab. 2008;93(8):3117-23.
- Gomez-Sanchez CE, Qi X, Gomez-Sanchez EP, Sasano H, Bohlen MO, Wisgerhof M. Disordered zonal and cellular CYP11B2 enzyme expression in familial hyperaldosteronism type 3. Molecular and cellular endocrinology. 2017;439:74-80.
- Zilbermint M, Xekouki P, Faucz FR, Berthon A, Gkourogianni A, Schernthaner-Reiter MH, et al. Primary Aldosteronism and ARMC5 Variants. J Clin Endocrinol Metab. 2015;100(6):E900-9.
- Dutta RK, Soderkvist P, Gimm O. Genetics of primary hyperaldosteronism. Endocrine-related cancer. 2016;23(10):R437-54.
- Korah HE, Scholl UI. An Update on Familial Hyperaldosteronism. Hormone and metabolic research = Hormon- und Stoffwechselforschung = Hormones et metabolisme. 2015;47(13):941-6.
- Scholl UI, Goh G, Stolting G, de Oliveira RC, Choi M, Overton JD, et al. Somatic and germline CACNA1D calcium channel mutations in aldosterone-producing adenomas and primary aldosteronism. Nature genetics. 2013;45(9):1050-4.
- Scholl UI, Stolting G, Nelson-Williams C, Vichot AA, Choi M, Loring E, et al. Recurrent gain of function mutation in calcium channel CACNA1H causes early-onset hypertension with primary aldosteronism. eLife. 2015;4:e06315.
- Rich GM, Ulick S, Cook S, Wang JZ, Lifton RP, Dluhy RG. Glucocorticoid-remediable aldosteronism in a large kindred: clinical spectrum and diagnosis using a characteristic biochemical phenotype. Annals of internal medicine. 1992;116(10):813-20.
- Ganguly A, Grim CE, Weinberger MH. Anomalous postural aldosterone response in glucocorticoid-suppressible hyperaldosteronism. N Engl J Med. 1981;305(17):991-3.
- Stowasser M, Gordon RD. Familial hyperaldosteronism. The Journal of steroid biochemistry and molecular biology. 2001;78(3):215-29.
- Ulick S, Chu MD. Hypersecretion of a new corticosteroid, 18-hydroxycortisol in two types of adrenocortical hypertension. Clinical and experimental hypertension Part A, Theory and practice. 1982;4(9-10):1771-7.
- Ulick S, Chu MD, Land M. Biosynthesis of 18-oxocortisol by aldosterone-producing adrenal tissue. The Journal of biological chemistry. 1983;258(9):5498-502.
- Litchfield WR, New MI, Coolidge C, Lifton RP, Dluhy RG. Evaluation of the dexamethasone suppression test for the diagnosis of glucocorticoid-remediable aldosteronism. J Clin Endocrinol Metab. 1997;82(11):3570-3.
- New MI, Peterson RE, Saenger P, Levine LS. Evidence for an unidentified ACTH-induced steroid hormone causing hypertension. J Clin Endocrinol Metab. 1976;43(6):1283-93.
- Jamieson A, Inglis GC, Campbell M, Fraser R, Connell JM. Rapid diagnosis of glucocorticoid suppressible hyperaldosteronism in infants and adolescents. Archives of disease in childhood. 1994;71(1):40-3.
- Stowasser M, Gordon RD. Primary aldosteronism: learning from the study of familial varieties. J Hypertens. 2000;18(9):1165-76.
- Fardella CE, Pinto M, Mosso L, Gomez-Sanchez C, Jalil J, Montero J. Genetic study of patients with dexamethasone-suppressible aldosteronism without the chimeric CYP11B1/CYP11B2 gene. J Clin Endocrinol Metab. 2001;86(10):4805-7.
- Stowasser M, Gartside MG, Gordon RD. A PCR-based method of screening individuals of all ages, from neonates to the elderly, for familial hyperaldosteronism type I. Australian and New Zealand journal of medicine. 1997;27(6):685-90.
- Jochmanova I, Pacak K. Pheochromocytoma: The First Metabolic Endocrine Cancer. Clinical cancer research : an official journal of the American Association for Cancer Research. 2016;22(20):5001-11.
- Eisenhofer G, Keiser H, Friberg P, Mezey E, Huynh TT, Hiremagalur B, et al. Plasma metanephrines are markers of pheochromocytoma produced by catechol-O-methyltransferase within tumors. J Clin Endocrinol Metab. 1998;83(6):2175-85.
- Plouin PF, Amar L, Dekkers OM, Fassnacht M, Gimenez-Roqueplo AP, Lenders JW, et al. European Society of Endocrinology Clinical Practice Guideline for long-term follow-up of patients operated on for a phaeochromocytoma or a paraganglioma. Eur J Endocrinol. 2016;174(5):G1-G10.
- Lenders JW, Duh QY, Eisenhofer G, Gimenez-Roqueplo AP, Grebe SK, Murad MH, et al. Pheochromocytoma and paraganglioma: an endocrine society clinical practice guideline. J Clin Endocrinol Metab. 2014;99(6):1915-42.
- Majumdar S, Friedrich CA, Koch CA, Megason GC, Fratkin JD, Moll GW. Compound heterozygous mutation with a novel splice donor region DNA sequence variant in the succinate dehydrogenase subunit B gene in malignant paraganglioma. Pediatric blood & cancer. 2010;54(3):473-5.
- Moramarco J, El Ghorayeb N, Dumas N, Nolet S, Boulanger L, Burnichon N, et al. Pheochromocytomas are diagnosed incidentally and at older age in neurofibromatosis type 1. Clinical endocrinology. 2016.
- Aufforth RD, Ramakant P, Sadowski SM, Mehta A, Trebska-McGowan K, Nilubol N, et al. Pheochromocytoma Screening Initiation and Frequency in von Hippel-Lindau Syndrome. J Clin Endocrinol Metab. 2015;100(12):4498-504.
- Varoquaux A, Kebebew E, Sebag F, Wolf K, Henry JF, Pacak K, et al. Endocrine tumors associated with the vagus nerve. Endocrine-related cancer. 2016;23(9):R371-9.
- de Wailly P, Oragano L, Rade F, Beaulieu A, Arnault V, Levillain P, et al. Malignant pheochromocytoma: new malignancy criteria. Langenbeck's archives of surgery. 2012;397(2):239-46.
- Lenders JW, Eisenhofer G, Mannelli M, Pacak K. Phaeochromocytoma. Lancet. 2005;366(9486):665-75.
- Motta-Ramirez GA, Remer EM, Herts BR, Gill IS, Hamrahian AH. Comparison of CT findings in symptomatic and incidentally discovered pheochromocytomas. AJR American journal of roentgenology. 2005;185(3):684-8.
- Shao Y, Chen R, Shen ZJ, Teng Y, Huang P, Rui WB, et al. Preoperative alpha blockade for normotensive pheochromocytoma: is it necessary? J Hypertens. 2011;29(12):2429-32.
- Eisenhofer G, Peitzsch M. Laboratory evaluation of pheochromocytoma and paraganglioma. Clinical chemistry. 2014;60(12):1486-99.
- Peaston RT, Graham KS, Chambers E, van der Molen JC, Ball S. Performance of plasma free metanephrines measured by liquid chromatography-tandem mass spectrometry in the diagnosis of pheochromocytoma. Clinica chimica acta; international journal of clinical chemistry. 2010;411(7-8):546-52.
- Lenders JW, Pacak K, Walther MM, Linehan WM, Mannelli M, Friberg P, et al. Biochemical diagnosis of pheochromocytoma: which test is best? JAMA. 2002;287(11):1427-34.
- Taylor RL, Singh RJ. Validation of liquid chromatography-tandem mass spectrometry method for analysis of urinary conjugated metanephrine and normetanephrine for screening of pheochromocytoma. Clinical chemistry. 2002;48(3):533-9.
- Waguespack SG, Rich T, Grubbs E, Ying AK, Perrier ND, Ayala-Ramirez M, et al. A current review of the etiology, diagnosis, and treatment of pediatric pheochromocytoma and paraganglioma. J Clin Endocrinol Metab. 2010;95(5):2023-37.
- Singer J, Koch CA, Kassahun W, Lamesch P, Eisenhofer G, Kluge R, et al. A patient with a large recurrent pheochromocytoma demonstrating the pitfalls of diagnosis. Nature reviews Endocrinology. 2011;7(12):749-55.
- Osinga TE, van der Horst-Schrivers AN, van Faassen M, Kerstens MN, Dullaart RP, Pacak K, et al. Mass spectrometric quantification of salivary metanephrines-A study in healthy subjects. Clinical biochemistry. 2016;49(13-14):983-8.
- Lenders JW, Willemsen JJ, Eisenhofer G, Ross HA, Pacak K, Timmers HJ, et al. Is supine rest necessary before blood sampling for plasma metanephrines? Clinical chemistry. 2007;53(2):352-4.
- Darr R, Pamporaki C, Peitzsch M, Miehle K, Prejbisz A, Peczkowska M, et al. Biochemical diagnosis of phaeochromocytoma using plasma-free normetanephrine, metanephrine and methoxytyramine: importance of supine sampling under fasting conditions. Clinical endocrinology. 2014;80(4):478-86.
- Boot C, Toole B, Johnson SJ, Ball S, Neely D. Single-centre study of the diagnostic performance of plasma metanephrines with seated sampling for the diagnosis of phaeochromocytoma/paraganglioma. Annals of clinical biochemistry. 2016.
- Sawka AM, Prebtani AP, Thabane L, Gafni A, Levine M, Young WF, Jr. A systematic review of the literature examining the diagnostic efficacy of measurement of fractionated plasma free metanephrines in the biochemical diagnosis of pheochromocytoma. BMC endocrine disorders. 2004;4(1):2.
- Eisenhofer G, Lenders JW, Timmers H, Mannelli M, Grebe SK, Hofbauer LC, et al. Measurements of plasma methoxytyramine, normetanephrine, and metanephrine as discriminators of different hereditary forms of pheochromocytoma. Clinical chemistry. 2011;57(3):411-20.
- Eisenhofer G, Goldstein DS, Walther MM, Friberg P, Lenders JW, Keiser HR, et al. Biochemical diagnosis of pheochromocytoma: how to distinguish true- from false-positive test results. J Clin Endocrinol Metab. 2003;88(6):2656-66.
- Bravo EL, Tarazi RC, Fouad FM, Vidt DG, Gifford RW, Jr. Clonidine-suppression test: a useful aid in the diagnosis of pheochromocytoma. N Engl J Med. 1981;305(11):623-6.
- Sjoberg RJ, Simcic KJ, Kidd GS. The clonidine suppression test for pheochromocytoma. A review of its utility and pitfalls. Arch Intern Med. 1992;152(6):1193-7.
- Sebel EF, Hull RD, Kleerekoper M, Stokes GS. Responses to glucagon in hypertensive patients with and without pheochromocytoma. The American journal of the medical sciences. 1974;267(6):337-43.
- Algeciras-Schimnich A, Preissner CM, Young WF, Jr., Singh RJ, Grebe SK. Plasma chromogranin A or urine fractionated metanephrines follow-up testing improves the diagnostic accuracy of plasma fractionated metanephrines for pheochromocytoma. J Clin Endocrinol Metab. 2008;93(1):91-5.
- Miehle K, Kratzsch J, Lenders JW, Kluge R, Paschke R, Koch CA. Adrenal incidentaloma diagnosed as pheochromocytoma by plasma chromogranin A and plasma metanephrines. J Endocrinol Invest. 2005;28(11):1040-2.
- Eisenhofer G, Huysmans F, Pacak K, Walther MM, Sweep FC, Lenders JW. Plasma metanephrines in renal failure. Kidney international. 2005;67(2):668-77.
- Sarathi V, Lila AR, Bandgar TR, Menon PS, Shah NS. Pheochromocytoma and pregnancy: a rare but dangerous combination. Endocrine practice : official journal of the American College of Endocrinology and the American Association of Clinical Endocrinologists. 2010;16(2):300-9.
- Neary NM, King KS, Pacak K. Drugs and pheochromocytoma--don't be fooled by every elevated metanephrine. N Engl J Med. 2011;364(23):2268-70.
- Welch TJ, Sheedy PF, 2nd, van Heerden JA, Sheps SG, Hattery RR, Stephens DH. Pheochromocytoma: value of computed tomography. Radiology. 1983;148(2):501-3.
- Lumachi F, Tregnaghi A, Zucchetta P, Cristina Marzola M, Cecchin D, Grassetto G, et al. Sensitivity and positive predictive value of CT, MRI and 123I-MIBG scintigraphy in localizing pheochromocytomas: a prospective study. Nuclear medicine communications. 2006;27(7):583-7.
- Blake MA, Krishnamoorthy SK, Boland GW, Sweeney AT, Pitman MB, Harisinghani M, et al. Low-density pheochromocytoma on CT: a mimicker of adrenal adenoma. AJR American journal of roentgenology. 2003;181(6):1663-8.
- Jacques AE, Sahdev A, Sandrasagara M, Goldstein R, Berney D, Rockall AG, et al. Adrenal phaeochromocytoma: correlation of MRI appearances with histology and function. European radiology. 2008;18(12):2885-92.
- Bhatia KS, Ismail MM, Sahdev A, Rockall AG, Hogarth K, Canizales A, et al. 123I-metaiodobenzylguanidine (MIBG) scintigraphy for the detection of adrenal and extra-adrenal phaeochromocytomas: CT and MRI correlation. Clinical endocrinology. 2008;69(2):181-8.
- Scholz T, Eisenhofer G, Pacak K, Dralle H, Lehnert H. Clinical review: Current treatment of malignant pheochromocytoma. J Clin Endocrinol Metab. 2007;92(4):1217-25.
- Solanki KK, Bomanji J, Moyes J, Mather SJ, Trainer PJ, Britton KE. A pharmacological guide to medicines which interfere with the biodistribution of radiolabelled meta-iodobenzylguanidine (MIBG). Nuclear medicine communications. 1992;13(7):513-21.
- Mozley PD, Kim CK, Mohsin J, Jatlow A, Gosfield E, 3rd, Alavi A. The efficacy of iodine-123-MIBG as a screening test for pheochromocytoma. Journal of nuclear medicine : official publication, Society of Nuclear Medicine. 1994;35(7):1138-44.
- Janssen I, Chen CC, Millo CM, Ling A, Taieb D, Lin FI, et al. PET/CT comparing (68)Ga-DOTATATE and other radiopharmaceuticals and in comparison with CT/MRI for the localization of sporadic metastatic pheochromocytoma and paraganglioma. European journal of nuclear medicine and molecular imaging. 2016;43(10):1784-91.
- Janssen I, Chen CC, Taieb D, Patronas NJ, Millo CM, Adams KT, et al. 68Ga-DOTATATE PET/CT in the Localization of Head and Neck Paragangliomas Compared with Other Functional Imaging Modalities and CT/MRI. Journal of nuclear medicine : official publication, Society of Nuclear Medicine. 2016;57(2):186-91.
- Archier A, Varoquaux A, Garrigue P, Montava M, Guerin C, Gabriel S, et al. Prospective comparison of (68)Ga-DOTATATE and (18)F-FDOPA PET/CT in patients with various pheochromocytomas and paragangliomas with emphasis on sporadic cases. European journal of nuclear medicine and molecular imaging. 2016;43(7):1248-57.
- Castinetti F, Kroiss A, Kumar R, Pacak K, Taieb D. 15 YEARS OF PARAGANGLIOMA: Imaging and imaging-based treatment of pheochromocytoma and paraganglioma. Endocrine-related cancer. 2015;22(4):T135-45.
- Melcescu E, Phillips J, Moll G, Subauste JS, Koch CA. 11Beta-hydroxylase deficiency and other syndromes of mineralocorticoid excess as a rare cause of endocrine hypertension. Hormone and metabolic research = Hormon- und Stoffwechselforschung = Hormones et metabolisme. 2012;44(12):867-78.
- Paperna T, Gershoni-Baruch R, Badarneh K, Kasinetz L, Hochberg Z. Mutations in CYP11B1 and congenital adrenal hyperplasia in Moroccan Jews. J Clin Endocrinol Metab. 2005;90(9):5463-5.
- Reisch N, Hogler W, Parajes S, Rose IT, Dhir V, Gotzinger J, et al. A diagnosis not to be missed: nonclassic steroid 11beta-hydroxylase deficiency presenting with premature adrenarche and hirsutism. J Clin Endocrinol Metab. 2013;98(10):E1620-5.
- Biglieri EG, Herron MA, Brust N. 17-hydroxylation deficiency in man. The Journal of clinical investigation. 1966;45(12):1946-54.
- Goldsmith O, Solomon DH, Horton R. Hypogonadism and mineralocorticoid excess. The 17-hydroxylase deficiency syndrome. N Engl J Med. 1967;277(13):673-7.
- Imai T, Yanase T, Waterman MR, Simpson ER, Pratt JJ. Canadian Mennonites and individuals residing in the Friesland region of The Netherlands share the same molecular basis of 17 alpha-hydroxylase deficiency. Human genetics. 1992;89(1):95-6.
- Costa-Santos M, Kater CE, Auchus RJ, Brazilian Congenital Adrenal Hyperplasia Multicenter Study G. Two prevalent CYP17 mutations and genotype-phenotype correlations in 24 Brazilian patients with 17-hydroxylase deficiency. J Clin Endocrinol Metab. 2004;89(1):49-60.
- Ulick S, Levine LS, Gunczler P, Zanconato G, Ramirez LC, Rauh W, et al. A syndrome of apparent mineralocorticoid excess associated with defects in the peripheral metabolism of cortisol. J Clin Endocrinol Metab. 1979;49(5):757-64.
- Wilson RC, Krozowski ZS, Li K, Obeyesekere VR, Razzaghy-Azar M, Harbison MD, et al. A mutation in the HSD11B2 gene in a family with apparent mineralocorticoid excess. J Clin Endocrinol Metab. 1995;80(7):2263-6.
- Mune T, Rogerson FM, Nikkila H, Agarwal AK, White PC. Human hypertension caused by mutations in the kidney isozyme of 11 beta-hydroxysteroid dehydrogenase. Nature genetics. 1995;10(4):394-9.
- Pizzolo F, Friso S, Morandini F, Antoniazzi F, Zaltron C, Udali S, et al. Apparent Mineralocorticoid Excess by a Novel Mutation and Epigenetic Modulation by HSD11B2 Promoter Methylation. J Clin Endocrinol Metab. 2015;100(9):E1234-41.
- Wilson RC, Nimkarn S, New MI. Apparent mineralocorticoid excess. Trends in endocrinology and metabolism: TEM. 2001;12(3):104-11.
- White PC, Agarwal AK, Nunez BS, Giacchetti G, Mantero F, Stewart PM. Genotype-phenotype correlations of mutations and polymorphisms in HSD11B2, the gene encoding the kidney isozyme of 11beta-hydroxysteroid dehydrogenase. Endocrine research. 2000;26(4):771-80.
- Knops NB, Monnens LA, Lenders JW, Levtchenko EN. Apparent mineralocorticoid excess: time of manifestation and complications despite treatment. Pediatrics. 2011;127(6):e1610-4.
- Lavery GG, Ronconi V, Draper N, Rabbitt EH, Lyons V, Chapman KE, et al. Late-onset apparent mineralocorticoid excess caused by novel compound heterozygous mutations in the HSD11B2 gene. Hypertension. 2003;42(2):123-9.
- Morineau G, Sulmont V, Salomon R, Fiquet-Kempf B, Jeunemaitre X, Nicod J, et al. Apparent mineralocorticoid excess: report of six new cases and extensive personal experience. Journal of the American Society of Nephrology : JASN. 2006;17(11):3176-84.
- Wang LP, Yang KQ, Jiang XJ, Wu HY, Zhang HM, Zou YB, et al. Prevalence of Liddle Syndrome Among Young Hypertension Patients of Undetermined Cause in a Chinese Population. Journal of clinical hypertension. 2015;17(11):902-7.
- Kellenberger S, Gautschi I, Rossier BC, Schild L. Mutations causing Liddle syndrome reduce sodium-dependent downregulation of the epithelial sodium channel in the Xenopus oocyte expression system. The Journal of clinical investigation. 1998;101(12):2741-50.
- Yang KQ, Lu CX, Xiao Y, Liu YX, Jiang XJ, Zhang X, et al. A novel frameshift mutation of epithelial sodium channel beta-subunit leads to Liddle syndrome in an isolated case. Clinical endocrinology. 2015;82(4):611-4.
- Cui Y, Tong A, Jiang J, Wang F, Li C. Liddle syndrome: clinical and genetic profiles. Journal of clinical hypertension. 2016.
- Nesterov V, Krueger B, Bertog M, Dahlmann A, Palmisano R, Korbmacher C. In Liddle Syndrome, Epithelial Sodium Channel Is Hyperactive Mainly in the Early Part of the Aldosterone-Sensitive Distal Nephron. Hypertension. 2016;67(6):1256-62.
- Canessa CM, Schild L, Buell G, Thorens B, Gautschi I, Horisberger JD, et al. Amiloride-sensitive epithelial Na+ channel is made of three homologous subunits. Nature. 1994;367(6462):463-7.
- Kashif Nadeem M, Ling C. Liddle's-like syndrome in the elderly. Journal of clinical hypertension. 2012;14(10):728.
- Tapolyai M, Uysal A, Dossabhoy NR, Zsom L, Szarvas T, Lengvarszky Z, et al. High prevalence of liddle syndrome phenotype among hypertensive US Veterans in Northwest Louisiana. Journal of clinical hypertension. 2010;12(11):856-60.
- Glover M, O'Shaughnessy KM. Molecular insights from dysregulation of the thiazide-sensitive WNK/SPAK/NCC pathway in the kidney: Gordon syndrome and thiazide-induced hyponatraemia. Clinical and experimental pharmacology & physiology. 2013;40(12):876-84.
- Ohta A, Schumacher FR, Mehellou Y, Johnson C, Knebel A, Macartney TJ, et al. The CUL3-KLHL3 E3 ligase complex mutated in Gordon's hypertension syndrome interacts with and ubiquitylates WNK isoforms: disease-causing mutations in KLHL3 and WNK4 disrupt interaction. The Biochemical journal. 2013;451(1):111-22.
- Nobel YR, Lodish MB, Raygada M, Rivero JD, Faucz FR, Abraham SB, et al. Pseudohypoaldosteronism type 1 due to novel variants of SCNN1B gene. Endocrinology, diabetes & metabolism case reports. 2016;2016:150104.
- Geller DS, Rodriguez-Soriano J, Vallo Boado A, Schifter S, Bayer M, Chang SS, et al. Mutations in the mineralocorticoid receptor gene cause autosomal dominant pseudohypoaldosteronism type I. Nature genetics. 1998;19(3):279-81.
- Geller DS, Zhang J, Zennaro MC, Vallo-Boado A, Rodriguez-Soriano J, Furu L, et al. Autosomal dominant pseudohypoaldosteronism type 1: mechanisms, evidence for neonatal lethality, and phenotypic expression in adults. Journal of the American Society of Nephrology : JASN. 2006;17(5):1429-36.
- Geller DS, Farhi A, Pinkerton N, Fradley M, Moritz M, Spitzer A, et al. Activating mineralocorticoid receptor mutation in hypertension exacerbated by pregnancy. Science. 2000;289(5476):119-23.
- New MI, Geller DS, Fallo F, Wilson RC. Monogenic low renin hypertension. Trends in endocrinology and metabolism: TEM. 2005;16(3):92-7.
- Ullah MI, Washington T, Kazi M, Tamanna S, Koch CA. Testosterone deficiency as a risk factor for cardiovascular disease. Hormone and metabolic research = Hormon- und Stoffwechselforschung = Hormones et metabolisme. 2011;43(3):153-64.
- Ullah MI, Uwaifo GI, Nicholas WC, Koch CA. Does vitamin d deficiency cause hypertension? Current evidence from clinical studies and potential mechanisms. International journal of endocrinology. 2010;2010:579640.