ABSTRACT
Ambiguous genitalia in a newborn are the clinical sign of atypical sexual development of the external genitalia in utero. This condition is rare and can result from various underlying factors, including certain disorders with potentially severe consequences, such as cortisol deficiency due to congenital adrenal hyperplasia. Therefore, it is crucial to promptly determine etiology when ambiguity is observed. The formation of typical male or female external genitalia is a complex process involving a cascade of genetic and physiological events that begin with sex determination and progress through the differentiation of internal and external reproductive structures. When this process is disrupted and does not occur in the typical manner, it is referred to as a difference or disorder of sex development (DSD). Not all DSD cases present with ambiguous genitalia at birth; for example, complete androgen insensitivity syndrome does not, but all cases of ambiguous genitalia are the result of a DSD. This chapter focuses on genital ambiguity associated with DSD in newborns who have either a 46,XY or 46,XX chromosomal sex. However, DSD with genital ambiguity may also be observed in newborns with other combinations of sex chromosomes, such as 45,X/46,XY. The chapter offers a comprehensive overview of the evaluation and management of newborns with ambiguous genitalia. It emphasizes the importance of a structured medical assessment of the external genitalia to diagnose and determine the underlying cause of genital ambiguity. It includes tables for differential diagnosis and step-by-step workup algorithms to guide medical professionals in their evaluation. It also includes additional information on structured physical assessments of external genitalia and tables with normative values for hormonal measurements, which are recommended in the diagnostic process. As the etiology of genital ambiguity in newborns is diverse and can have significant implications for management, the authors stress that obtaining an accurate diagnosis through a professional medical workup is crucial. The chapter highlights the recommendation for newborns with ambiguous genitalia (DSD) to be cared for by highly specialized, interdisciplinary DSD teams. These teams are equipped with medical and psychosocial expertise, and specialized psychologists are available to support parents and caregivers. The chapter recognizes that having a child with ambiguous genitalia can be very stressful for parents. It underscores the importance of early education, access to expert care through DSD network teams, psychological support, shared decision-making, and promoting acceptance and inclusivity. By providing comprehensive support and guidance, families can better navigate the challenges and uncertainties they may encounter when caring for a newborn with ambiguous genitalia.
INTRODUCTION
Typical male and female development commences with the presence of the typical 46,XY or 46,XX chromosomes, which play a pivotal role in determining the indifferent gonads. Around the 6th week of gestation, these gonads follow pathways to develop into male-typical or female-typical gonads (Figure 1). This gonadal determination is intricately regulated by a complex interplay of multiple genes, which guide the male gonads to become testes and the female gonads to become ovaries.
Subsequently, the gonads initiate the production of various hormones, including anti-Müllerian hormone (AMH) and testosterone. These hormones are responsible for driving the differentiation of the embryologic Wolffian and Müllerian structures into sex-typical internal reproductive organs (Figure 1).
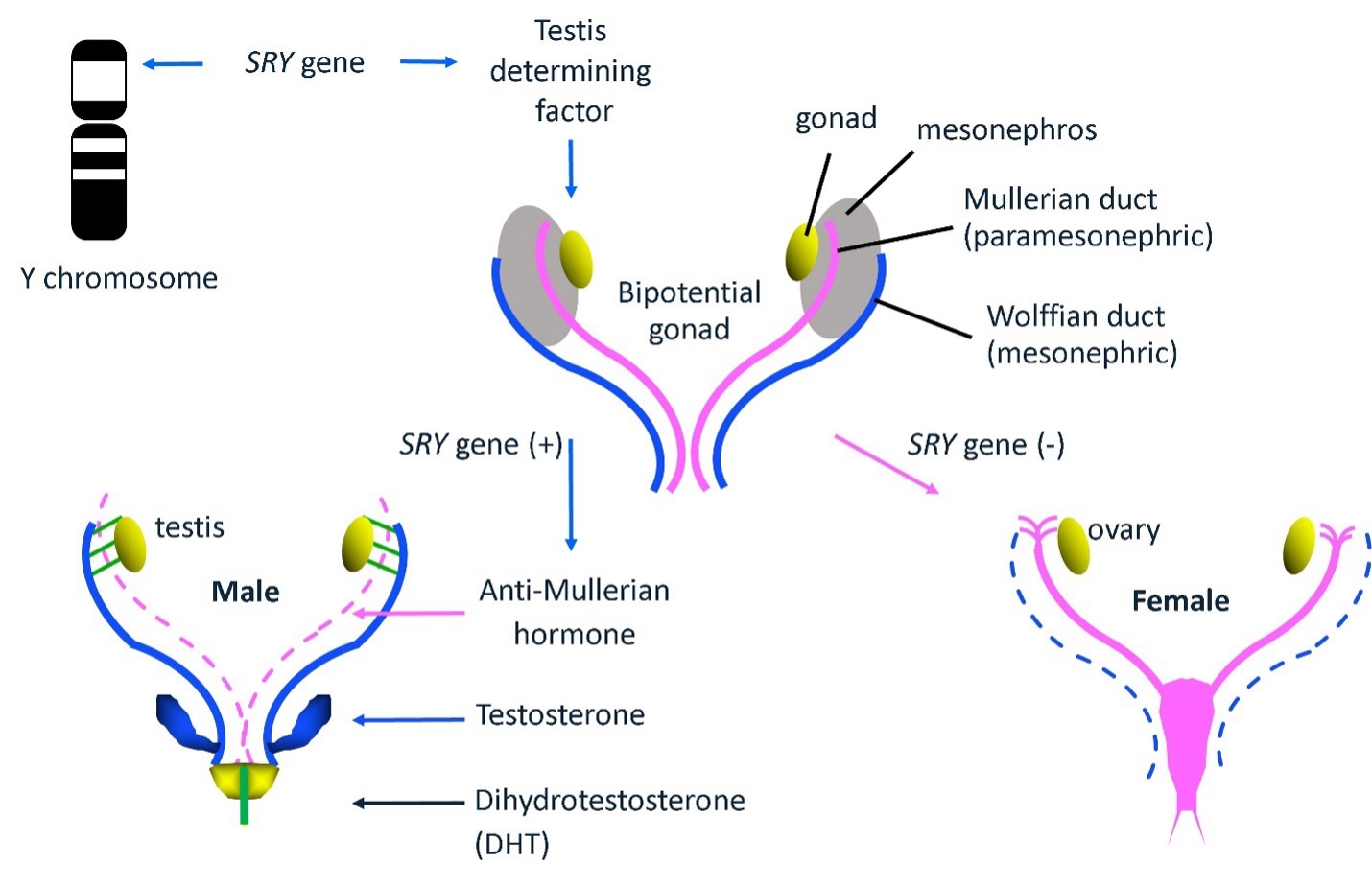
Figure 1. Typical male and female sex determination and differentiation occur during fetal life. The presence of the Y chromosome in the karyotype and the SRY gene (sex-determining region Y gene) are the primary determinants of male sex development. The SRY gene initiates a cascade that leads to the development of testes from bipotential gonads. Testosterone and Anti Mullerian Hormone (AMH) are secreted by testicular cells, which masculinize the Wolffian (mesonephric) duct and cause the regression of the Mullerian (paramesonephric) duct, respectively. Dihydrotestosterone (DHT), produced by the 5-alpha reduction of testosterone, plays a major role in male external genital development and prostate formation. The absence of the SRY gene on the Y chromosome is the primary factor for the induction of the female sex, and the development of the uterus, fallopian tubes, cervix and upper vagina from the Mullerian duct.
The development of external genitalia begins with a neutral anlage, including a genital tubercule, genital folds, and a urogenital sinus. These structures are subsequently differentiated into typical male external genitalia under the influence of androgens, particularly dihydrotestosterone (DHT). Conversely, for the development of typical female external genitalia, the absence of androgens is crucial to prevent virilization.
Therefore, ambiguous genitalia at birth can arise from either an excessive or insufficient androgen effect on the neutral external genital anlage in males or females, respectively (Figure 2).
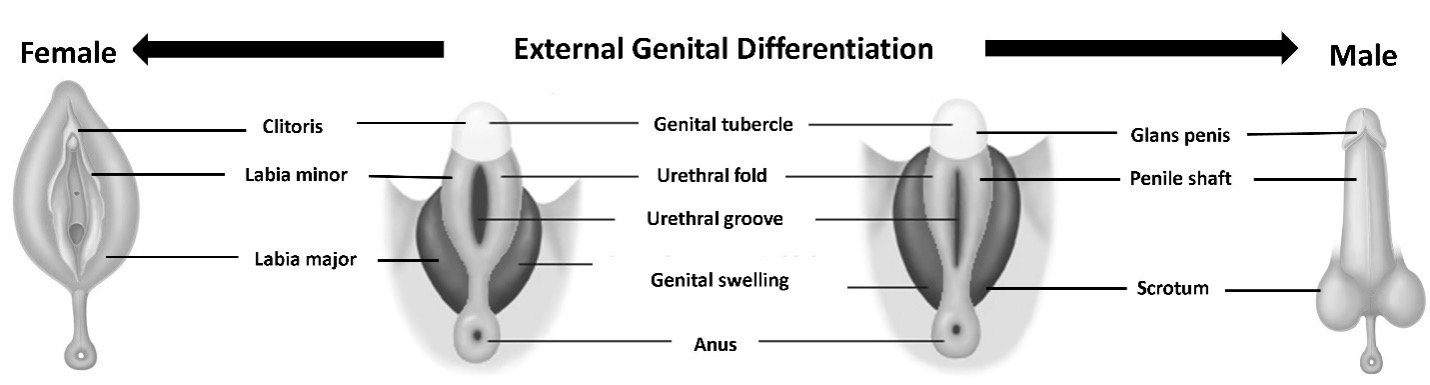
Figure 2. External genital differentiation. The development of external genitalia begins with a bipotential anlage which includes genital tubercle, urethral fold, urethral groove, and genital swellings. In a typical 46, XY, androgen secretion from the testes triggers the fusion of urethral folds, allowing the enclosure of the urethral tube. This, together with the cells from the genital swelling, forms the shaft of the penis. Genital swellings fuse in the midline to allow formation of the scrotum and genital Tubercle expands to give rise to the glans penis. Female external genital development is ensured by the absence of testosterone, it is independent of ovarian endocrine activity. Urethral folds and genital swellings remain separate to form the labia minora and majora. Genital tubercle forms the clitoris.
CHARACTERISTICS OF TYPICAL MALE AND FEMALE EXTERNAL GENITALIA
A full-term male infant is typically expected to have bilateral testicles that are descended, complete formation of scrotal folds with midline fusion, and a typical-sized penis, which includes well-formed corporal bodies and a urethral meatus located at the tip. The average penile length for a full-term newborn is approximately 3.5 ± 0.4 cm, but this measurement can vary with gestational age (Table 1) (1, 2). If an infant presents with bilateral cryptorchidism, a bifid scrotum, hypospadias, or isolated penoscrotal hypospadias, further investigations for a DSD are recommended. However, it’s important to note that isolated micropenis, as long as both testes are descended and normal in size, is not typically considered a manifestation of ambiguous genitalia. Similarly, in males, distal hypospadias with no other atypical genital features usually does not indicate a DSD (3).
Table 1. Stretched Penile Length in Preterm and Term Newborns According to Gestational Age (2)
|
Gestational age (week)
|
Number of cases
|
Median (cm)
|
Mean (cm)
|
SD
|
Min (cm)
|
Max (cm)
|
26
|
30
|
1.9
|
1.9
|
0.32
|
1.1
|
2.5
|
27
|
31
|
2.1
|
2.0
|
0.28
|
1.1
|
2.6
|
28
|
32
|
2.0
|
1.9
|
0.31
|
1.3
|
2.9
|
29
|
29
|
2.3
|
2.3
|
0.39
|
1.3
|
2.9
|
30
|
31
|
2.4
|
2.4
|
0.32
|
1.6
|
3.0
|
31
|
33
|
2.4
|
2.4
|
0.38
|
1.8
|
3.3
|
32
|
32
|
2.9
|
2.6
|
0.44
|
2.0
|
3.2
|
33
|
28
|
2.9
|
2.8
|
0.45
|
1.9
|
3.4
|
34
|
30
|
2.9
|
2.8
|
0.43
|
2.0
|
3.6
|
35
|
29
|
3.1
|
2.9
|
0.61
|
2.0
|
4.1
|
36
|
31
|
3.1
|
3.0
|
0.50
|
2.2
|
4.0
|
37
|
29
|
3.1
|
3.0
|
0.47
|
2.2
|
4.1
|
38
|
44
|
3.1
|
3.1
|
0.54
|
2.1
|
4.5
|
39
|
82
|
3.2
|
3.2
|
0.55
|
2.0
|
4.2
|
40
|
65
|
3.5
|
3.4
|
0.53
|
2.2
|
4.4
|
41
|
29
|
3.6
|
3.5
|
0.47
|
2.6
|
4.3
|
A full-term female infant typically presents with bilateral separated labial folds, no palpable gonads, and distinct urethral and vaginal openings (Figure 2). The average clitoral length and width for a full-term infant girl are approximately 6.1 mm and 4.2 mm, respectively (1, 4). In cases where clitoromegaly (clitoral size ˃10 mm), labial fusion or palpable gonads are observed in otherwise typical female genitalia, further investigation for a DSD is warranted. It’s important to note that perceived clitoromegaly is not typically associated with an underlying DSD in the event that the newborn girl was born prematurely (1). Please be aware that perceived clitoromegaly or pseudo-clitoromegaly is often seen in preterm newborns, where the clitoral size falls within the normal range, but the labia majora cannot completely cover labia minora, creating a misleading impression of clitoromegaly. Genital edema may also be observed in sick preterm babies or cases of ovarian hyperstimulation, which can cause pseudo-clitoromegaly.
Table 2. Clitoral Length and Width in Preterm and Term Newborns According to Gestational Age (4)
|
Gestational age (week)
|
Number of cases
|
Measure
|
Mean (mm)
|
+1 SD (mm)
|
+2 SD (mm)
|
+3 SD (mm)
|
28-30
|
30
|
Width
|
3.28
|
3.87
|
4.46
|
5.05
|
Length
|
5.03
|
5.91
|
6.79
|
7.67
|
30-32
|
57
|
Width
|
3.32
|
3.68
|
4.04
|
4.4
|
Length
|
5.03
|
5.52
|
6.01
|
6.5
|
32-34
|
74
|
Width
|
3.41
|
3.77
|
4.13
|
4.49
|
Length
|
5.14
|
5.65
|
6.16
|
6.67
|
34-36
|
104
|
Width
|
3.69
|
4.17
|
4.65
|
5.13
|
Length
|
5.53
|
6.12
|
6.71
|
7.3
|
36-38
|
128
|
Width
|
4.08
|
4.5
|
4.92
|
5.34
|
Length
|
5.83
|
6.29
|
6.75
|
7.21
|
>38
|
187
|
Width
|
4.21
|
4.64
|
5.07
|
5.5
|
Length
|
6.11
|
6.49
|
6.87
|
7.25
|
GENERAL THOUGHTS ON AMBIGUOUS GENITALIA AT BIRTH
The reported incidence of ambiguous genitalia in newborns is approximately one in 4,500. It is essential that a newborn with ambiguous genitalia and their parents are promptly referred to a specialized center for evaluation. This evaluation should be carried out by a multi-disciplinary team specializing in DSD in accordance with international recommendations on diagnostic and therapeutic strategies. This team should provide holistic care and treatment guidance, collaborating closely with relevant subspecialists and peer support groups (5). Key subspecialties involved in DSD teams typically include psychology, pediatric endocrinology, urology, neonatology, gynecology, andrology, nursing, social work, genetics, and medical ethics. It's worth noting that certain aspects of DSD management remain contentious or uncertain, resulting in recent changes in clinical practice that are ongoing. Notably, genital surgery has been banned in several countries, and it is increasingly avoided in young children to protect their rights to an open future and the integrity of their bodies.
Consequently, we can anticipate a growing number of children growing up with atypical-looking genitalia, requiring a careful assessment of the psychological impact (6). Providing psychological support to affected families as a standard component of care from the outset is, therefore, of utmost importance. Issues related to gender that may arise in the context of ambiguous genitalia should be openly and thoughtfully discussed.
A comprehensive evaluation of ambiguous genitalia in a newborn is essential for understanding the underlying cause and potential consequences. On one hand, this evaluation can lead to the detection of concomitant, treatable disorders, such as adrenal insufficiency that requires cortisol replacement. Also, it is important to note that in up to 30% of children with a Difference/Disorder of Sex Development (DSD), other organ system disorders may be identified, necessitating medical care and qualifying as syndromic DSD (7, 8). On the other hand, identifying the underlying cause of ambiguous genitalia forms the basis for making an informed decision regarding sex registration at birth, if necessary and desired by the parents. Gender assignment/sex registration at birth should take into account long-term satisfaction with the sex of rearing, sexual function, and fertility potential (1, 9, 10). Ideally, it should result from a shared decision-making process involving the child's parents and the DSD network team. This decision should be based on the individual's phenotype/genotype, existing knowledge from literature, databases, and patient representative experience (11-13). This process may require time, as parents need to grasp the complexity of their child's unique biology in order to participate in this decision. As a first step, the overall health of the child should be assessed, and parents should be educated about typical sexual development before explanations regarding the underlying cause and its implications for their child's ambiguous genitalia are provided. Even if the exact details of the underlying cause remain unresolved, it is essential to communicate what is known and what remains uncertain to the parents and make plans for the family's future in the best interests of the child.
Several websites are available for patients and their families to exchange information, share coping strategies, and discuss decision-making processes that can enhance outcomes for newborns with ambiguous genitalia. Such resources can be found on websites supported by organizations like the Accord Alliance (www.accordalliance.org), DSD families (www.dsdfamilies.org), AIS DSD Support Group (www.aisdsd.org), and the CARES Foundation (www.caresfoundation.org). However, the most current information will always be available from the responsible DSD team, which will also be aware of country-specific support services.
GENETICS OF SEX DETERMINATION AND DIFFERENTIATION
Sex development is a sequential process that involves the coordinated action of numerous genes and pathways. This process culminates in the development of functional gonads, differentiated internal sex organs, external genitalia, and typical secondary sexual characteristics that manifest after puberty. Prenatal sex development can be divided into two distinct processes: sex determination and sex differentiation (Figure 3). Sex determination is the initial step that occurs shortly after conception, approximately at 6-7 weeks gestation. During this phase, the undifferentiated gonads evolve into either testes or ovaries. By contrast, sex differentiation occurs subsequently and involves hormones produced after the formation of the sex-specific gonads. These hormones play a critical role in shaping the further development of the dependent embryonic structures, leading to the formation of male and female internal and external phenotypes.
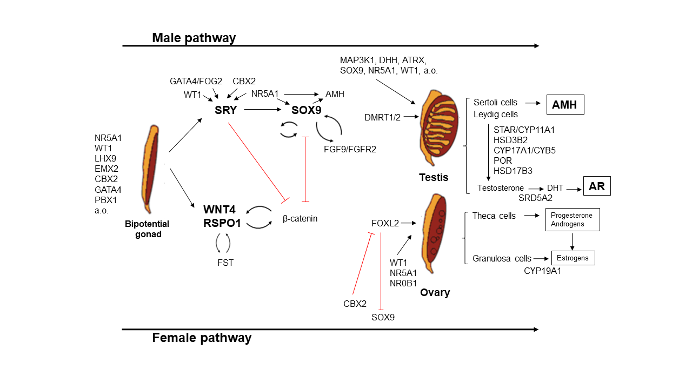
Figure 3. The genes and transcription factors required for gonadal development. The formation of the bipotential gonad requires at least seven genes including NR5A1 (Nuclear Receptor Subfamily 5 Group A Member 1), WT1 (WilmsTumor1 Transcription Factor), LHX9 (LIM Homeobox 9), EMX2 (Empty Spiracles Homeobox 2), CBX2 (Chromobox 2), PBX1 (Pre-B-Cell Leukemia Transcription Factor 1) and GATA4 (GATA Binding Protein 4). Adapted from (14).
The Male Specific Pathway
In the male specific pathway, the expression of the SRY gene in pre-Sertoli cells serves as a pivotal switch that directs the fate of the gonads toward testicular development. SRY, in conjunction with NR5A1, activates the SOX9 signaling pathway, initiating testis development (Figure 3). Other important genes for the early testis development are NR0B1(Nuclear Receptor subfamily 0 group B member 1), AMH (Anti-Mullerian Hormone) and GATA4. AMH secreted by Sertoli cells, plays a crucial role in promoting the regression of Müllerian structures and supporting the development of Leydig cells in the testes. Subsequently, androgens produced by the testes facilitate the development of male internal organs (epididymis, vas deferens, seminal vesicle) and external genitalia (penis, scrotum). The production of sex steroids relies on an intact steroidogenic pathway, with all steroid hormones originating from cholesterol. The initial steps of sex steroid biosynthesis overlap with the synthesis of mineralo-corticosteroids and gluco-corticosteroids (Figure 4). It is important to note that certain steroid disorders, often referred to as congenital adrenal hyperplasia (CAH), can affect both adrenal and gonadal steroidogenesis or result in androgen excess or deficiency that may impact fetal genital development. INSL3, produced in Leydig cells, plays a role in guiding the descent of the testes from the abdomen to the scrotal folds.
The process of masculinization of initially sex-neutral, undifferentiated external genitalia begins around 8-9 weeks of gestation when the potent androgen DHT (dihydrotestosterone) is produced by the testes. DHT is crucial for the fusion of the urethral and labioscrotal folds, elongation of the genital tubercle, regression of the urogenital sinus, and the development of the prostate (15). The enzyme 5α-reductase is responsible for converting testosterone produced by fetal Leydig cells into DHT. Complete masculinization of the external genitalia through DHT is typically achieved by week 14 of gestation (16).
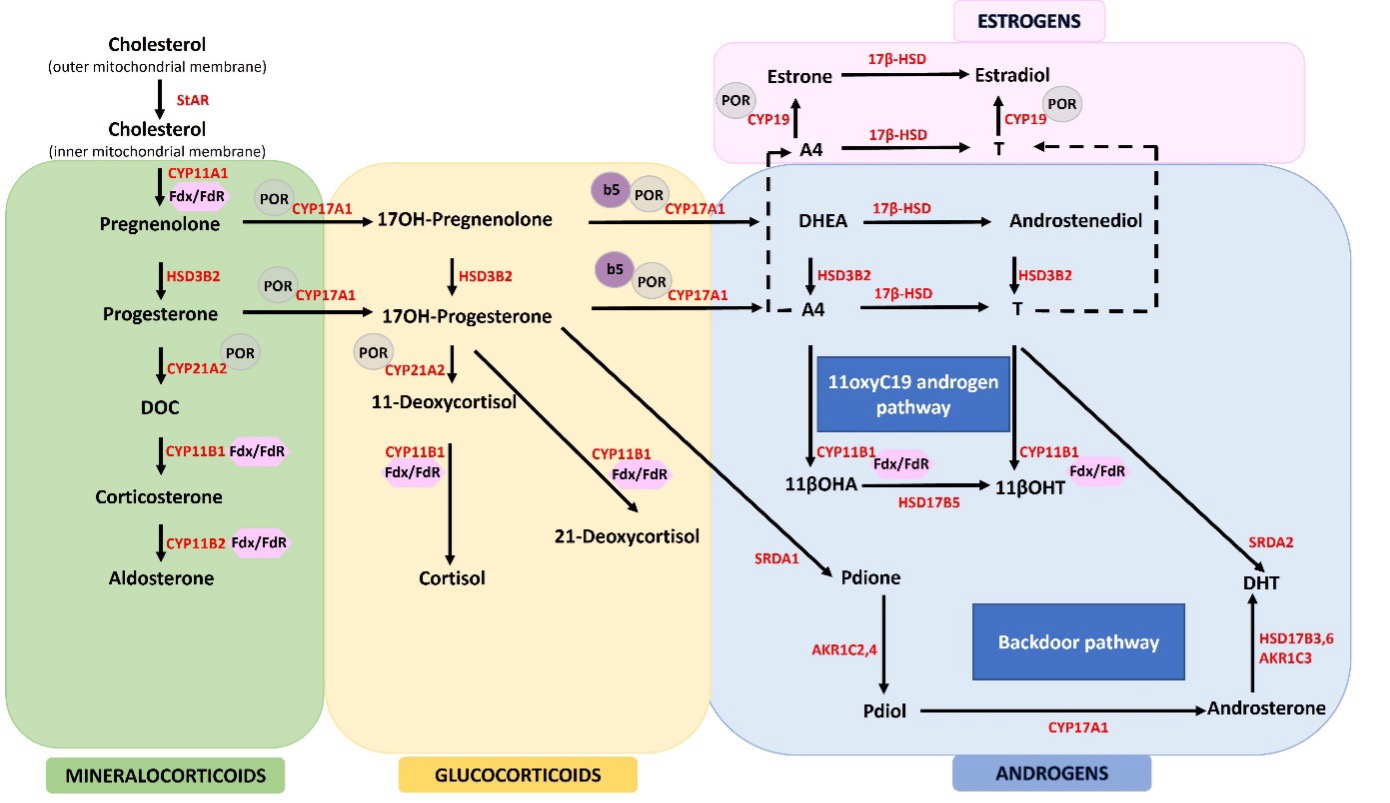
Figure 4. Steroidogenesis of the adrenals and gonads. Adrenal and gonadal steroidogenesis starting from cholesterol and showing key steroids and intermediates of the classical and alternative pathways is illustrated. Cortisol and androgen biosynthesis are under control of hypothalamic-pituitary-adrenal (HPA) axis in a negative feedback loop. Androgen synthesis occurs via classical or aberrant and alternative pathways (i.e., backdoor pathway and 11oxC19 androgen pathway). Aberrant or alternative pathways are particularly active whenever cortisol with or without aldosterone biosynthesis is impaired and androgenic precursors accumulate proximal to enzymatic block. Abbreviations: StAR; Steroidogenic Acute Regulatory Protein defect, CYP11A1; P450 side-chain cleavage deficiency, CYP21A2; 21α-hydroxylase, HSD3B2; 3β-hydroxy steroid dehydrogenase 2, CYP11B1; 11β-hydroxylase, CYP11B2; Aldosterone synthase, DOC; 11-deoxycorticosterone, DHEA; Dehydroepiandrosterone, A4; Androstenedione, T; Testosterone, CYP17A1; 17α-hydroxylase/17,20-lyase, b5; CYP19A1; Cytochrome P450 aromatase, Cytochrome b5, POR; P450 oxidoreductase, 17β-HSD; 17β-hydroxysteroid oxidoreductase or 17-ketosteroid reductase; SRDA1/2; Steroid 5α -reductase 1/2, 11oxC19; 11-oxygenated 19-carbon (11oxC19) steroids; Fdx; Ferredoxin, FdR; Ferredoxin reductase, 11βOHA; 11β-hydroxyandrostenedione, 11βOHT; 11β-hydroxytestosterone, Pdione; 5α-Pregnane- 17α-ol-3,20-dione, Pdiol; 5α-Pregnane- 3α,17α-diol 20-one, AKR1C2,4; Aldo-Keto Reductase Family 1 Member C2,4, DHT; Dihydrotestosterone, HSD17B5,3,6= 17β-hydroxysteroid dehydrogenase 5,3,6. Adapted from (17).
The Female Specific Pathway
By contrast, the female-specific pathway is initiated in the absence of SRY and requires the suppression of SOX9 (Figure 3). Activation of Rspo1/Wnt4/b-catenin and Foxl2 (Forkhead Box L2) signaling pathways leads to the formation of typical ovaries. The absence of AMH allows the Müllerian structures to proliferate, resulting in the formation of the typical fallopian tube, uterus, cervix and the upper part of the vagina. In the absence of both testosterone secretion and 5α-reductase activity, the sex-neutral external genitalia develop along the female pathway. Specifically, without DHT, the labioscrotal and urethral folds give rise to the labia majora and minora, respectively. The genital tubercle develops into a clitoris, and the urogenital sinus contributes to the formation of the urethral opening and anterior portion of the vagina (16).
Any disruption in the intricate genetic and hormonal processes involved can lead to atypical sex development and result in atypical external genitalia at birth. Figure 3 provides a summary of these processes and highlights some key genes and hormones essential for typical development.
DIFFERENCES/DISORDERS OF SEX DEVELOPMENT (DSD)
The discordance between genetic, gonadal, or anatomic sex is commonly referred to as DSD. In addition to candidate gene testing, the new era of molecular diagnostic tools, including whole exome/genome sequencing has uncovered numerous novel molecular etiologies in recent years. Accurate molecular diagnosis aids in managing affected individuals and provides families with information concerning prognosis and the risk of recurrence. This information is often sourced from literature and comprehensive data registries, such as the I-DSD (18-24). For clinical purposes, DSD (including ambiguous genitalia in newborns) is typically classified based on the affected individual's karyotype. The consensus statement addressing the approach and care of DSD (originally published in 2006 and updated in 2016) suggests the following broad classification for DSD: (A) Sex Chromosome DSD, (B) 46,XY DSD, and (C) 46,XX DSD (Table 3) (1, 20).
Table 3. DSD Classification According to the Chicago Consensus.
|
Sex Chromosome DSD
|
46,XY DSD
|
46,XX DSD
|
A. 45,X0 (Turner syndrome and variants)
|
A. Disorders of gonadal (testicular) development
Complete gonadal dysgenesis (Swyer syndrome) or partial gonadal dysgenesis (e.g., SRY,SOX9, NR5A1, WT1, DHHgene mutations etc)
Testicular regression (e.g., DHX37 gene mutation)
Ovo-Testicular DSD
|
A. Disorder of gonadal (ovarian) development
Ovo-Testicular DSD
Testicular DSD (SRY (+))
Gonadal dysgenesis
|
B. 47,XXY (Klinefelter syndrome and variants)
|
B. Disorders of testicular hormone production or action
Impaired testosterone production
LH receptor mutations
CAH (3βHSD2 deficiency, 17OHD, POR, StAR, CYP11A1 deficiency)
HSD17B3 deficiency
Impaired testosterone action
5α-reductase deficiency
Androgen insensitivity syndrome (Complete/partial)
Impaired AMH production or action
Persistent Mullerian Duct Syndrome (AMH and AMHR2gene mutations)
|
B. Androgen excess
Fetal
CAH (21-hydroxylase, 11β-hydroxylase, 3βHSD2 deficiency)
Glucocorticoid resistance
Feto-placental
Aromatase deficiency
POR deficiency
Maternal
Luteoma, hilar cell tumors, arrhenoblastoma, lipoid cell tumors, Krukenberg tumors, androgen producing adrenal tumors,
External androgen exposure
|
C. 45,X0/46,XY (Mixed gonadal dysgenesis, ovotesticular DSD)
|
C. Other
Syndromic
Smith-Lemli-Opitz, Cloacal anomaly, Aarskog, Robinow, Meckel, Joubert, Hand-Foot-Genital, popliteal pterygium, CHARGE, VATER/VACTERL, IMAGe etc.
Drugs
Flutamide, ketoconazole, progestagens
Endocrine disruptors
Phthalate, BPA, paraben
Cryptorchidism
INSL3, GREAT gene mutations etc.
Isolated hypospadias
MAMLD1, HOXA4 gene mutations etc.
|
C. Other
Syndromic
Cloacal anomaly etc.
Mullerian anomalies
Mayer-Rokitanski-Küster-Hauser, MURCS, McKusick-Kaufmnann syndrome and variants, MODY5
|
D. 46,XX/46,XY (chimeric, ovotesticular DSD)
|
|
|
Abbreviations: StAR; Steroidogenic Acute Regulatory Protein defect, CYP11A1; P450 side-chain cleavage deficiency, 3βHSD2; 3β-hydroxy steroid dehydrogenase 2, CYP17A1; 17α-hydroxylase/17,20-lyase deficiency, 17OHD; 17α-hydroxylase/17,20-lyase deficiency, POR; P450 oxidoreductase, HSD17B3; 17β-hydroxysteroid oxidoreductase or 17-ketosteroid reductase; SF1/NR5A1; steroidogenic factor 1.
The 46,XY Newborn With Ambiguous Genitalia
Ambiguous genitalia in a 46XY newborn can result from abnormal formation of the early fetal testes (testicular dysgenesis), reduced production of testosterone or dihydrotestosterone (5α-reductase deficiency), or the inability to respond to androgens (androgen insensitivity syndrome, or AIS) (Table 3). Depending on the extent of androgen production defect or resistance, affected newborns may exhibit a range of external genitalia phenotype, varying from those that appear typically female to those that appear typically male but with a small phallus, hypospadias, and a bifid scrotum, with or without palpable testes. Here we focus primarily on causes that lead to ambiguous genitalia. Broadly, four groups of underlying causes of 46, XY DSD with ambiguous genitalia can be identified: (a) partial gonadal dysgenesis, (b) partial testosterone biosynthetic defects, (c) partial 5α-reductase deficiency, and (d) partial androgen insensitivity syndrome or PAIS.
PARTIAL GONADAL DYSGENESIS
In cases of partial gonadal dysgenesis, it is presumed that a gene mutation leads to a partial abnormality in the development of the early urogenital ridge or gonadal anlage. This category of DSD can also involve mutations in genes such as SRY and SOX9, which are essential for the differentiation of bipotential gonads into testes (Figure 3). Partial gonadal dysgenesis is typically associated with incomplete masculinization of the external genitalia, along with varying degrees of maintenance of Wolffian ducts and inhibition of Müllerian ducts. For a summary of known genetic variations associated with gonadal dysgenesis, please refer to Table 4.
Leydig cell aplasia or hypoplasia represents a variation of gonadal dysgenesis, characterized by inadequate Leydig cell differentiation in the male gonad, resulting in impaired androgen production. Mutations in the LH receptor gene (LHCGR) as well as polymorphisms have been associated with this condition (25). The phenotype associated with Leydig cell hypoplasia includes incomplete masculinization of the external genitalia, along with incomplete development and maintenance of the Wolffian ducts.
Table 4. Monogenic Disorders of Gonadal Development/Differentiation and Associated DSD Presentations in Humans, Both 46,XY and 46,XX
|
Gene defect
|
Locus
|
Mode of transmission
|
Clinical presentation
|
Reference
|
|
|
|
DSD phenotype
|
Other
|
|
|
|
|
46, XX
|
46, XY
|
|
|
ARX
|
Xp22.13
|
XL
|
|
Testicular dysgenesis
|
Lissencephaly, epilepsy, intellectual disability temperature instability
|
(26)
|
ATRX
|
Xq13.3
|
XL
|
|
Testicular dysgenesis
|
Dysmorphic features, intellectual disability, α-thalassemia
|
(27)
|
CBX2
|
17q25
|
AR
|
Ovarian dysgenesis
|
Testicular dysgenesis
|
|
(28)
|
DHH
|
12q13.12
|
AR
|
|
Testicular dysgenesis
|
Minifascicular neuropathy
|
(29)
|
DMRT1/DMRT2
|
9p24.3
|
AD
|
Ovarian dysgenesis
|
Testicular dysgenesis
|
Hypotonia, developmental delay, impaired intellectual development
|
(30)
|
DHX37
|
12q24.31
|
AD
|
|
Testicular dysgenesis, testicular regression
|
|
(31)
|
EMX2
|
10q26.11
|
|
Ovarian dysgenesis
|
Testicular dysgenesis
|
Intellectual disability, kidney agenesis
|
(32)
|
ESR2
|
14q23.2-q23.3
|
AD,?
|
Ovarian dysgenesis
|
Testicular dysgenesis
|
Dysmorphic features, eye abnormalities, anal atresia, rectovestibular fistula
|
(33)
|
FGFR2
|
10q26.13
|
AD
|
|
Testicular dysgenesis, ovotesticular
|
Craniosynostosis
|
(34)
|
GATA4
|
8p23.1
|
AD
|
|
Testicular dysgenesis
|
Congenital heart defects (atrial septum defects, ventricular septum defects, tetralogy of Fallot), diaphragmatic hernia
|
(35)
|
HHAT
|
1q32.2
|
AR
|
|
Testicular dysgenesis
|
Nivelon-Nivelon-Mabille syndrome. Dwarfism, chondrodysplasia, narrow, bell-shaped thorax, micromelia, brachydactyly, microcephaly with cerebellar vermis hypoplasia, facial anomalies, hypoplastic irides and coloboma of the optic discs
|
(36)
|
LHX9
|
1q31.3
|
AD
|
|
Testicular dysgenesis
|
Limb anomalies
|
(37)
|
MAMLD1
|
Xq28
|
XL
|
|
Severe hypospadias
|
Myotubular myopathy
|
(38)
|
MAP3K1
|
5q11.2
|
AD
|
|
Testicular dysgenesis
|
|
(39)
|
NR0B1 (Duplications, inversion
and upstream deletion)
|
X21.3
|
XL
|
|
Testicular dysgenesis
|
Congenital adrenal hypoplasia, hypogonadotropic hypogonadism, cleft palate, intellectual disability
|
(40)
|
NR2F2
|
15q26.2
|
AD
|
Ovarian dysgenesis, ovotesticular DSD
|
|
Congenital heart disease, somatic anomalies including
blepharophimosis-ptosis-epicanthus
inversus syndrome
|
(41)
|
NR5A1
|
9q33
|
AD
|
Ovarian dysgenesis, ovotesticular DSD
|
Testicular dysgenesis
|
Adrenocortical insufficiency,
spermatogenic failure, primary ovarian insufficiency, asplenia, polysplenia
|
(42)
|
PPP2R3C
|
14q13.2
|
AR
|
|
Testicular dysgenesis
|
Dysmorphic facies, retinal dystrophy, and
myopathy.
|
(43)
|
RSPO1
|
1p34.3
|
AR
|
Testicular/
ovotesticular DSD
|
|
Palmoplantar hyperkeratosis
|
(44)
|
SART3
|
12q23.3
|
AR
|
|
Testicular dysgenesis
|
Intellectual disability, global developmental delay and a subset of brain anomalies
|
(45)
|
SOX3 (Upstream deletion and
Duplication)
|
Xq27.1
|
XL
|
Testicular/ovotesticular DSD
|
|
Intellectual disability, hypopituitarism
|
(46)
|
SOX8 (SNV, upstream
duplication, inversion)
|
16p13.3
|
AD
|
|
Testicular dysgenesis
|
Variants may contribute to male and female infertility, primary ovarian insufficiency.
|
(47)
|
SOX9 (SNV, upstream
duplication, inversion)
|
17q24.3
|
AD
|
Testicular/ovotesticular DSD
|
Testicular dysgenesis
|
Campomelic dysplasia, Cooks syndrome, Pierre Robin sequence
|
(48)
|
SOX10 (Duplication)
|
22q13.1
|
AD
|
Testicular/ovotesticular DSD
|
|
Peripheral and central demyelination, Waardenburg syndrome type IV, and Hirschsprung disease
|
(49)
|
SRY (SNV, deletion,
Translocation)
|
Yp11.3
|
XL, YL
|
Testicular/ovotesticular DSD
|
Testicular dysgenesis
|
|
(50, 51)
|
TSPYL1
|
6q22.1
|
AR
|
|
Testicular dysgenesis
|
Sudden infant death syndrome
|
(52)
|
WT1
|
11p13
|
AD
|
Testicular/ovotesticular DSD
|
Testicular dysgenesis
|
Denys-Drash syndrome; Frasier syndrome; WAGR syndrome; Meacham syndrome, Nephrotic syndrome; Wilms tumor
|
(53, 54)
|
Abbreviations: AD; autosomal dominant, AR; autosomal recessive, XL; X-linked, YL; Y-linked
DEFICIENCY OF TESTOSTERONE BIOSYNTHESIS
The inability to produce testosterone stems from defects in the activity of any of the enzymes required for testosterone biosynthesis from cholesterol (Figure 4able 5). Identified defects encompass genetic variants in Steroidogenic Acute Regulatory Protein (StAR), P450 side-chain cleavage (CYP11A1), 3β-hydroxy steroid dehydrogenase 2 (HSD3B2), 17α-hydroxylase/17,20-lyase (CYP17A1), and P450 oxidoreductase (POR) (59). In addition, apparent lyase deficiency due to variants in cytochrome b5 (CYB5) and variants in AKR1C2/4 have been very rarely described (60-62). Moreover, 17β-hydroxysteroid dehydrogenase deficiency (also known as 17β–hydroxysteroid oxidoreductase or 17-ketosteroid reductase, HSD17B3) is another cause of 46,XY DSD (63). Furthermore, variants in steroidogenic factor 1 (SF1/NR5A1), which regulates the transcription of several genes involved in steroidogenesis, may lead to ambiguous genitalia. Notably, variants in HSD3B2, POR, and NR5A1 can result in ambiguous genitalia in both 46,XY and 46,XX newborns (64-66).
Similar to partial gonadal dysgenesis, a partial testosterone biosynthesis defect leads to ambiguous external genitalia and variable degrees of Wolffian duct development. However, unlike partial gonadal dysgenesis, Müllerian ducts are not retained in 46,XY newborns with partial testosterone biosynthesis defects due to intact Sertoli cell function and normal AMH production.
Table 5. Adrenal and/or Gonadal Causes of Impaired Sex Steroid Biosynthesis Associated with Ambiguous Genitalia in 46,XX and 46,XY Individuals
|
Disorder
|
Gene/ OMIM
|
Adrenal Insufficiency
|
46,XY Gonadal Phenotype
(Testosterone Deficiency)
|
46,XX Gonadal Phenotype
(E2 Deficiency)
|
Fertility
|
Other Features
|
Lipoid congenital adrenal hyperplasia (LCAH)
|
StAR
201710
|
YES
|
Classic form: 46,XY DSD, gonadal insufficiency; ambiguous genitalia
Non-classic form: normal or NK
|
Classic: primary or secondary ovarian insufficiency (POI)
Non-classic: NK or normal
|
Classic:Absent in 46,XY; variable in 46,XX
|
|
P450 side chain cleavage syndrome (CAH)
|
CYP11A1
118485
|
YES
|
Classic form: 46,XY DSD, gonadal insufficiency; ambiguous genitalia
Non-classic form: normal
|
Classic: primary or secondary ovarian insufficiency (POI)
Non-classic: NK or normal
|
Reported in 46,XX
|
|
3β-hydroxysteroid dehydrogenase II deficiency (CAH)
|
HSD3B2
201810
|
YES
|
46,XY DSD, gonadal insufficiency; ambiguous genitalia
Non-classic form: normal, but premature adrenarche
|
46,XX DSD with atypical genital development; gonadal insufficiency; ambiguous genitalia
Non-classic form: normal, but premature adrenarche
|
Absent in 46,XY; reported in 46,XX
|
|
21-hydroxylase deficiency (CAH)
|
CYP21A2
201910
|
YES
|
Classic form: normal
Non-classic form: normal
|
46,XX DSD with atypical genital development;
Non-classic form: premature adrenarche, virilization, PCO
|
Normal in both 46,XX and 46,XY, if treated
|
Cave: Testicular adrenal rest tumor (m>>f)
CAH-X (when combined with Ehlers-Danlos syndrome with contiguous gene variants)
|
11-hydroxylase deficiency (CAH)
|
CYP11B1
202010
|
YES
|
Classic form: normal
Non-classic form: normal
|
46,XX DSD with atypical genital development;
Non-classic form: premature adrenarche, virilization, PCO
|
Normal in both 46,XX and 46,XY, if treated
|
Hypertension
|
Combined 17-hydroxylase, 17,20 lyase deficiency (CAH)
|
CYP17A1
202110
|
Rare
|
46,XY DSD, gonadal insufficiency; atypical genital development
|
Lack of pubertal development, POI
|
Possible in 46,XX with assisted fertility measures
|
Hypertension and hypokalemic alkalosis (not seen with isolated lyase deficiency)
|
P450 oxidoreductase deficiency (CAH)
|
POR
124015
201750
|
Variable
|
Mild to severe 46,XY DSD, gonadal insufficiency; atypical genital development
|
46,XX DSD with atypical genital development or
premature adrenarche, virilisation, POI, PCO
|
Reported
|
Maternal virilization during pregnancy; Antley-Bixler skeletal malformation syndrome; changes in drug metabolism
|
Cytochrome b5 deficiency
|
CYB5A
613218
|
NO
|
46,XY DSD; atypical genital development
|
NK
|
NK
|
Methemoglobinemia
|
17β-hydroxysteroid dehydrogenase III deficiency / 17-ketosteroid reductase deficiency
|
HSD17B3
264300
|
NO
|
46,XY DSD; atypical genital development; progressive virilization and gynecomastia at puberty
|
Normal
|
Decreased or absent in 46,XY
|
|
5α-reductase II deficiency
|
SRD5A2
607306
|
NO
|
46,XY DSD; atypical genital development; progressive virilisation and gynecomastia at puberty
|
Normal
|
Impaired in 46,XY
|
|
3α-hydroxysteroid dehydrogenase deficiency
|
AKR1C2/4
600450
600451
|
NO
|
46,XY DSD; gonadal insufficiency; atypical genital development
|
Normal
|
NK
|
|
Aromatase deficiency
|
CYP19A1
107910
|
NO
|
Normal
|
46,XX DSD with variable degree of virilisation at birth (ambiguous genitalia), gonadal insufficiency, POI
|
Impaired in 46,XX
|
Overgrowth and metabolic anomalies in males
|
Steroidogenic factor 1
|
NR5A1/
SF1
184757
|
Rare
|
Mild to severe 46,XY DSD; gonadal insufficiency – very variable; ambiguous genitalia
|
POI or normal; rarely 46,XX DSD with atypical genital development
|
Mostly impaired in 46,XY; variable in 46,XX
|
|
Abbreviations: NK; not known, POI; primary ovarian insufficiency, PCO; polycystic ovary.
5-ALPHA-REDUCTASE-2 DEFICIENCY (SRD5A2 VARIANTS)
Deficiency of the 5α-reductase enzyme arises from variants in the steroid 5α-reductase type 2 (SRD5A2) gene, which can show single point mutations to complete gene deletions. Newborns affected by this condition possess functioning Leydig and Sertoli cells, but due to their inability to convert testosterone to DHT, they may exhibit varying degrees of under-masculinized external genitalia, including genital ambiguity at birth in some cases (16, 67-69). The Müllerian ducts in affected individuals regress as expected, due to normal Sertoli cell function and AMH production. During puberty, significant virilization becomes possible in affected individuals, and the testes are capable of supporting spermatogenesis, as DHT is not required for germ cell maturation. Therefore, fertility is attainable in less severely affected individuals (70-72) or with the use of intrauterine insemination. Recent evidence also suggests that SRD5A2 activity may be even influenced by genetic polymorphisms of SRD5A2 (50).
GENETIC DEFECTS OF ANDROGEN ACTION
Complete or partial androgen insensitivity syndromes (CAIS/PAIS) are caused by genetic mutations affecting androgen receptor (AR) function (73, 74), and serum testosterone levels are typically elevated. Internationally, incidence of AIS is reported in 1 in 20,400 live born 46,XY infants, with CAIS occurring at a higher rate than PAIS (75).
The human androgen receptor (AR) is encoded by a single gene (AR) composed of 8 exons located in the q11-12 region of the X chromosome. The AR gene mutation database includes hundreds of AR variants that lead in varying degrees of atypical sex differentiation of 46,XY fetuses, also classified as AIS type I (76-78). A small number of complete AR gene deletions have been reported, as well as deletions that start at exons 2, 3 or 4 and extend to the terminus of the gene. In addition, a limited number of mutations resulting from premature terminations, base deletions and terminations have been identified. However, the most common type of AR gene mutation results from base substitutions (79). When mutations result in partial inactivation of AR activity (PAIS), the phenotypic variability in under-masculinization can be substantial and depends on the residual activity of the AR. In some cases, this can lead to ambiguous genitalia in newborns with a 46,XY chromosomal sex.
Unlike in CAIS, the underlying genetic defect in PAIS is only found in the AR gene in about 40% of cases (80). However, the clinical and biochemical phenotype is similar, and in vitro functional tests demonstrate androgen resistance in all of them. Thus, individuals with AIS without AR mutations are classified as AIS type II (73). In cases of AIS type II without AR variants, largely unidentified regulators or cofactors of the AR are responsible for the impaired AR signaling, as revealed by an AR-dependent bioassay using genital skin fibroblasts and the targeted apolipoprotein D as a biomarker (81). Given that AR activity can be regulated at various levels, the potential mechanisms of AIS type II are diverse.Recently, altered DNA methylation of the AR promoter has been observed in some individuals with PAIS (82). Additionally, mutations in the Disheveled associated activator of morphogenesis 2 gene (DAAM2) that impair nuclear actin assembly at AR have been associated with AIS type II (83).
Overall, individuals with PAIS experience variable degrees of end-organ unresponsiveness to androgens, resulting in varying degrees of Wolffian duct development and external genital ambiguity. Within families with the same AR mutation, phenotypic variability can occur due to variable degrees of insensitivity to androgens (84, 85). Consequently, solely relying on genetic information, it is challenging to predict whether a newborn with PAIS will identify as male, intersex, or female in later life and respond to future testosterone therapy. Similarly, genetic counselling is challenging.
The 46,XX Newborn With Ambiguous Genitalia
Ambiguous genitalia in a chromosomal 46,XX fetus invariably results from androgen excess during fetal development, and the degree of virilization (Figure 2) often provides clues about the timing and severity of the underlying disorder.
Similar to a 46,XY fetus, a 46,XX fetus may experience partial gonadal dysgenesis or develop testicular or ovo-testicular DSD due to pathogenic variants in genes involved in early sex determination (Table 3 and 4). Ovo-testicular DSD occurs when both ovarian and testicular tissue develop in the same individual (1). Most newborns with ovo-testicular DSD possess 46,XX chromosomes and present with ambiguous genitalia, although some affected individuals have 46,XY chromosomes or 46,XX/46,XY mosaicism. Just like in 46,XY DSD, the degree of testicular development determines the extent of masculinization/virilization of the external genitalia, Wolffian duct development, and Müllerian duct regression in affected newborns (79). A summary of known genetic variations is given in Table 4.
In the majority of cases, ambiguous genitalia in a 46,XX fetus with typical ovarian organogenesis can be attributed to excessive androgen production/exposure early in utero, originating either from the fetus itself (Table 3 and Table 5) or from the mother or environment.
ABNORMAL FETAL ANDROGEN PRODUCTION BY CONGENITAL ADRENAL HYPERPLASIA (CAH)
The term congenital adrenal hyperplasia (CAH) encompasses several adrenal disorders, each linked to a mutation in one of the enzymes necessary for the biosynthesis of cortisol from cholesterol (86) (Figure 4 and Table 5). These abnormalities lead to increased ACTH secretion by the pituitary gland, which, in turn, results in the increased secretion of cortisol precursors, including adrenal androgens.
Deficiency of steroid acute regulatory protein (StAR) causes congenital lipoid adrenal hyperplasia, characterized by salt loss and a lack of cortisol, androgen, and estrogen secretion. Genetic 46,XX infants affected by StAR deficiency exhibit typical female external genitalia, while genetic 46,XY are born with ambiguous genitalia or may experience sex reversal in cases of severe loss-of-function variants. A similar phenotype is also observed with variants in CYP11A1.
Deficiency in 17α-hydroxylase/17,20-lyase leads to hypertension due to the hypersecretion of corticosterone and impaired androgen secretion. Similar to congenital lipoid adrenal hyperplasia, 46,XX fetus affected by 17α-hydroxylase/17, 20-lyase deficiency (CYP17A1) develop typical female external genitalia, while 46,XY fetus present with ambiguity.
Deficiency of 3β-hydroxysteroid dehydrogenase (HSD3B2) results in salt loss and impaired androgen synthesis. Affected 46,XX females may or may not exhibit minimal genital masculinization, whereas all genetic 46,XY males are variably under-masculinized (87).
CAH due to 11β-hydroxylase deficiency (CYP11B1) and 21-hydroxylase deficiency (CYP21A2) results in the most significant masculinization/virilization of external genitalia in 46,XX fetus compared to all other types of CAH. Additionally, 11β-hydroxylase deficiency can lead to hypertension in individuals of either sex in later life (88, 89).
21-hydroxylase deficiency CAH, caused by variants in CYP21A2, represents the most common form (accounting for more than 90% of cases) of CAH and is the most frequent genetic cause of DSD with ambiguous genitalia at birth in 46,XX newborns (59, 63). Cortisol deficiency necessitates replacement therapy in all classic forms of CAH. In the milder (simple-virilizing) presentation of classical 21-hydroxylase deficiency, salt loss is typically not an issue, whereas in the more severe (salt-wasting) form of classical 21-hydroxylase deficiency, salt-loss occurs and may pose a risk to the newborn shortly after birth if not treated with both mineralocorticoids and glucocorticoids (86, 90, 91).
The pathological mechanisms of 21-hydroxylase deficiency leading to 46,XX virilization in utero have been well described (92). It involves androgen excess due to lack of 21-hydroxylase activity during the critical time window around 6-12 weeks of gestation when the external genitalia are formed, and adrenal androgen production should be redirected to cortisol production to protect the female external genital anlage. In 46,XX CAH (Table 5), the resulting adrenal androgen excess exceeds the androgen metabolizing capacities of the fetal-placental unit, and alternative pathways of androgen production (Figure 4) are also activated, contributing to the overall androgen excess. Figure 5 illustrates in a scheme how the fetal adrenals, liver and placenta cooperate under normal conditions to metabolize fetal adrenal androgens into estrogens.
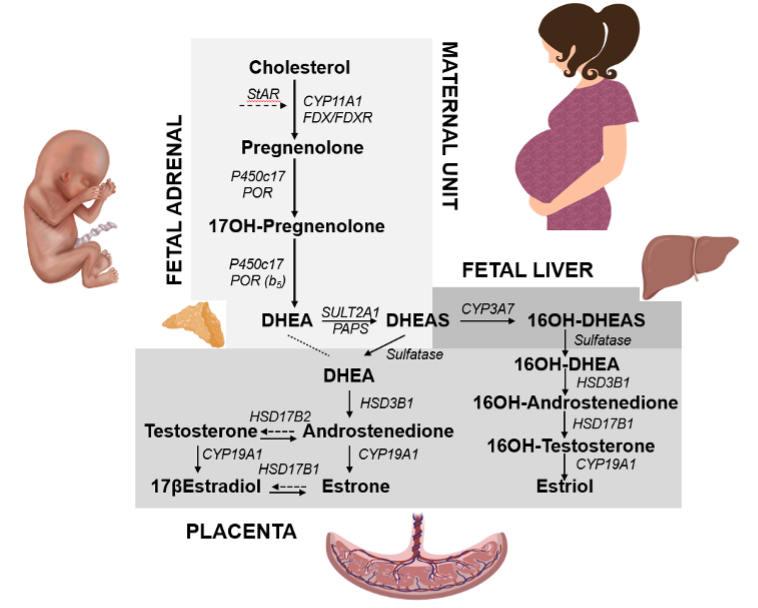
Figure 5. Steroid metabolic pathways in the fetal-placental-maternal unit, also known as the fetal-placental unit. The main function of the FZ of the fetal adrenal is the production of DHEA-S from cholesterol, which is transported to the placenta, desulfated to DHEA, and sequentially metabolized by placental 3β-hydroxysteroid dehydrogenase type 1 (3βHSD1), 17β-hydroxysteroid dehydrogenases (17βHSD) and cytochrome P450 aromatase (CYP19A1), to androstenedione (A4), testosterone (T) and estradiol (E2), respectively. E2 is then transported from the placenta into the maternal circulation. A4 is additionally also a substrate for placental CYP19A1, producing estrone (E1). Fetal adrenal DHEA-S is also metabolized by the fetal liver to 16α-hydroxy-DHEA-S by cytochrome P450 family 3 subfamily A member 7 (CYP3A7), which is metabolized to estriol (E3), the estrogen marker of pregnancy. Abbreviations: PREG, pregnenolone; 17OHPREG, 17α-hydroxypregnenolone; PROG, progesterone; DHEA, dehydroepiandrosterone; DHEA-S, dehydroepiandrosterone-sulfate; CYP11A1, cytochrome P450 cholesterol side chain cleavage; StAR, steroidogenic acute regulatory protein; CYP17A1, cytochrome P450 17α-hydroxylase/17,20-lyase; SULT2A1, sulfotransferase; CYP3A7, cytochrome P450 family 3 subfamily A member 7; CYP19A1, cytochrome P450 aromatase; HSD17B, 17β-hydroxysteroid dehydrogenase; HSD3B1, 3β-hydroxysteroid dehydrogenase type 1; HSD11B2, 11β-hydroxysteroid dehydrogenase type 2; STS, sulfatase.
FETAL AND PLACENTAL AROMATASE DEFICIENCY
During fetal development, the adrenals produce substantial amounts of DHEA/S. These are either converted to 16-hydroxy-DHEA/S in the fetal liver and then transported or directly transferred to the placenta, where they are converted into estrogens (Figure 5). In case of an aromatase enzyme deficiency in the fetus (caused by variants in the CYP19A1 gene), androgen precursors accumulate and lead to virilization of 46,XX fetuses, typically leading to ambiguous genitalia at birth (93). Androgen excess due to fetal aromatase deficiency may also induce maternal virilization during pregnancy, as indicated by a deepening of the voice, oily skin and hair, and excessive hair growth (hirsutism).
EXCESS MATERNAL ANDROGEN PRODUCTION
Excessive androgen production can negatively impact fertility due to anovulation, therefore cases of maternal androgen production during pregnancy are exceedingly rare. Nonetheless, androgens originating from the mother can travers the placenta and lead to the masculinization of a female fetus. These maternal androgens usually originate from the ovaries or adrenal glands. Androgen-producing ovarian tumors include hilar cell tumors, arrhenoblastomas/androblastomas, lipoid cell tumors, and Krukenberg tumors. In contrast, pregnancy luteomas, rare non-neoplastic ovarian lesions occurring during pregnancy, are believed to be caused by hormonal effects related to pregnancy. Although exceptionally rare, androgen-secreting tumors of the adrenals can also occur during pregnancy.
DRUGS ADMINISTERED TO THE MOTHER DURING GESTATION
In 1958, Wilkins et al. reported that certain synthetic progestins administered to pregnant women, such as 17α-ethinyl-19-nortestosterone, can masculinize the external genitalia of female fetuses (94). Similarly, diethylstilbesterol (DHE), a nonsteroidal synthetic estrogen, has been linked with urogenital anomalies in both 46,XX and 46,XY fetuses (95, 96). More recently, numerous studies have indicated that fetal exposure to various endocrine disrupting chemicals (EDCs) such as pesticides, fungicides, herbicides, and plasticizers may adversely affect genital development (97-99).
Syndromes Associated with Ambiguous Genitalia
As previously mentioned in sections of 46,XX and 46,XY DSD, ambiguous genitalia can be associated with syndromes characterized by multiple congenital malformations, as summarized in Table 4. These associations arise because many of the transcription factors involved in sex development and differentiation also have roles in other aspects of development (Table 4).
For example, mutations of the WT-1 gene (11p3) can lead to several syndromes, including WAGR, Denys-Drash or Frasier syndromes (70-72). Mutations in SOX-9 (17q24-25) can result in campomelic dysplasia (100).
Mutations in DMRT1/DMRT2 (9p24.3), EMX2 (10q25.3q26.13), ATRX (Xq13.3) and WNT-4 (1p35) are often associated with developmental delay (101, 102). SF-1 (9q33) and DAX-1 (Xp21.3) play roles in the early formation of the adrenals, anterior pituitary, and parts of the hypothalamus.
Consequently, mutations can lead to abnormalities of these organs, in addition to those of the urogenital system. Abnormal development of the lower abdominal wall with pubic diastasis can result in urogenital anomalies observed in the VA(C)TER(L) and CHARGE syndromes, as well as bladder and cloacal exstrophy (103).
These examples underscore the complexities of the anomalies that may be associated with ambiguous genitalia in newborns and emphasize the importance of conducting a thorough physical examination and involving an experienced DSD team when faced with a child presenting ambiguous genitalia.
WORKUP OF NEWBORNS WITH AMBIGUOUS GENITALIA
The etiology of genital ambiguity in newborns is diverse and can have significant implications for management. Therefore, obtaining an accurate diagnosis through a medical workup is crucial. Some investigations are urgent, such as ruling out or confirming and treating potentially life-threatening adrenal insufficiency with mineralocorticoid and glucocorticoid replacement therapy in children with underlying CAH. Additionally, associated organ anomalies may require immediate attention to ensure the child’s survival, such as cardiac, pulmonary, or kidney malfunctions.
When the newborn is shown to be capable of excreting urine and stool without problems, and adrenal and other organ functions are found to be intact, investigations to understand genital ambiguity become primarily a psychosocial “emergency” for the family and caregivers. As a result, psychological support and holistic care by a DSD team as early as possible are essential to guide the parents and make decisions in the child’s best long-term interest. Obtaining an accurate diagnosis of the underlying cause of genital ambiguity early on is necessary for decision-making processes regarded to sex registration, hormonal and surgical treatments, and outcomes such as potential fertility and risk of gonadal malignancy. Therefore, it is recommended practice that a thorough workup should be performed for every child born with ambiguous genitalia, and guidelines for such assessments are available (22, 104-107).
Overall Assessment of the Child with Ambiguous Genitalia
Figure 6 provides an overview of the initial workup for a newborn with ambiguous genitalia. Several aspects are considered, including pregnancy and family history, as well as postnatal adaptation (such as feeding, weight gain, alertness, excretion). In some cases, suspicion of genital ambiguity may arise during pregnancy through ultrasound surveillance. In such cases, first contact and information through a DSD team member (e.g., neonatologist, geneticist, pediatric endocrinologist) together with the DSD psychologist is recommended to guide the parents through pregnancy and alert the future care team early. Prenatal workup of ambiguous genitalia is generally not necessary if the fetus is otherwise developing normally without other organ anomalies that require attention. It is important to note that a fetus with CAH does not suffer from mineralocorticoid and cortisol deficiency in utero, as these hormones become essential only after birth (Figure 4). Genetic workup of a child with ambiguous genitalia during the prenatal period may only be recommended when there is a previous index case with a diagnosis, or when parental genetic data inform an abnormality, and when genetic counseling of the parents has led to a personalized action plan.
When taking the medical history of a newborn with ambiguous genitalia, asking specific questions can help guide the diagnostic workup and management. Figure 6 provides a list of important questions to consider. For example, androgenic drugs used in pregnancy may explain the virilization of a 46, XX newborns. Maternal virilization during pregnancy, for example, voice change and hirsutism may be important for the diagnoses of maternal virilizing tumors, P450 oxidoreductase (POR) deficiency, or placental aromatase deficiency. Questions about parental consanguinity are important, because many of the causes of ambiguous genitalia are recessively inherited. History of previously unexplained neonatal deaths, ambiguous genitalia, infertility or genital surgery in the family is important for identifying potential genetic etiologies.
In addition to the medical history, every newborn with ambiguous genitalia should undergo a comprehensive physical examination, with a special focus on signs of adrenal insufficiency (dehydration, lethargy, poor feeding) and any dysmorphic features (Figure 6). This examination may guide the diagnostic process.
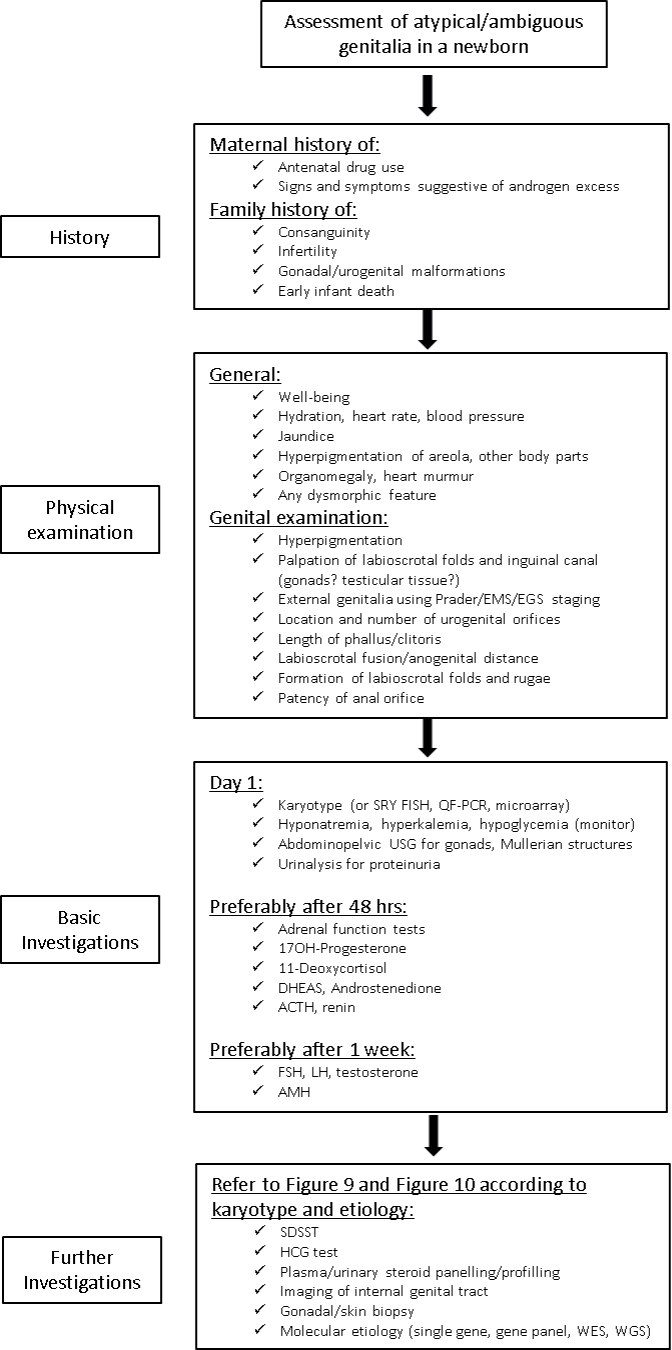
Figure 6. Algorithm for initial workup of the child with ambiguous genitalia at birth.
Abbreviations: SDSST; Standard dose synthetic ACTH (synacthen) stimulation test, HCG; Human chorionic gonadotropin, WES; Whole exome sequencing, WGS; Whole genome sequencing, EMS; External masculinization score, EGS; External genital score, QF-PCR; quantitative fluorescence polymerase chain reaction, SRY FISH; SRY fluorescent in situ hybridization.
Physical Examination of the External Genital Area
Systematic assessment of the external genital area in newborns with ambiguous genitalia is crucial, and it should be carefully documented. Over the years, different scales and scoring systems have been used to evaluate the external genitalia. These assessments help provide a standardized way to document the physical characteristics, which is essential for comparing data over time and between different healthcare providers and patients. The use of these scales and scoring systems aids in follow-up and tracking of changes in the external genitalia.
Historically, the Prader or Quigley scales were commonly used for this purpose (108, 109) (Figure 7). Later, the external masculinization score (EMS) was introduced as a more comprehensive assessment tool (104) (Table 6). Nowadays, the external genital score (EGS) is recommended for evaluating and documenting the external genitalia (110) (Table 7). These tools provide a structured way to assess and record the physical findings, making it easier to monitor and communicate about the patient's condition.
The careful examination of the external genitalia in newborns with ambiguous genitalia provides valuable non-invasive, indirect information about prenatal exposure and response to DHT, and about the timing of this exposure. For instance, androgen exposure must have occurred in the first trimester to cause labial fusion.
During the genital exam, it’s important to measure the stretched phallic length, assess the quality of the corpora (erectile tissues), inspect of the labia, labio-scrotal folds, or scrotum, and document the position of the urethral opening (and vaginal opening, if applicable). Furthermore, the presence and location of palpable gonads should also be recorded. Any asymmetry in the gonads and external genitalia should be noted. Asymmetry in internal and/or external genital structures may suggest mixed gonadal dysgenesis or ovo-testicular DSD. All these observations can be documented using the external genital score (EGS), which provides a standardized way to assess and record the physical characteristics of the external genitalia (Table 7). In addition, the anogenital distance (AGD), the distance between the anus and the base of the genitalia, can be helpful in determining the extent of androgen exposure during fetal development, as a higher ratio is generally consistent with increased androgen exposure (111) (Table 8). In older infants, the anogenital index (AGI), which is the body weight standardized index of AGD [AGI/weight (mm/kg)], may be used for assessments to account for changes of the external genitalia through growth and postnatal development (111).
Overall, a thorough examination and documentation of the external genitalia provide important clinical information that can guide the diagnosis and management of newborns with ambiguous genitalia.
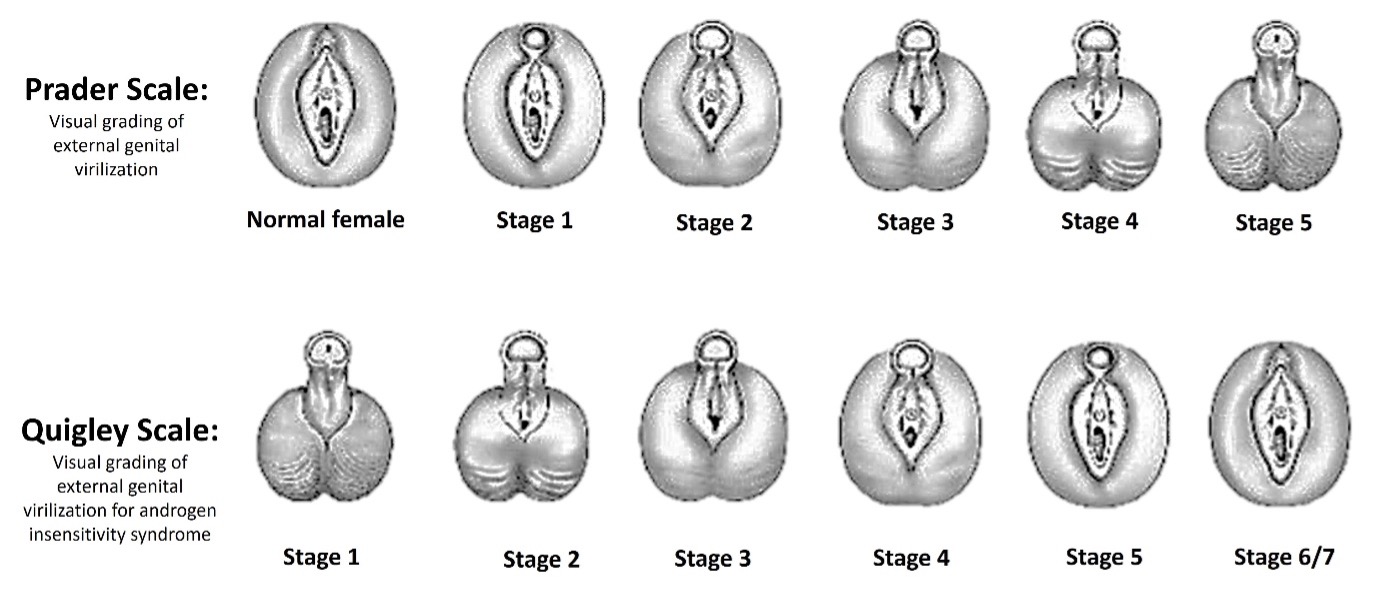
Figure 7. Prader and Quigley Scales for visual grading of external genital virilization. In Stage 3 a single urogenital orifice can be observed.
Table 6. External Masculinization Score (EMS)
|
External Masculinization Score (EMS).
|
Scrotal fusion
|
Micropenis
|
Urethral meatus
|
Right gonad
|
Left gonad
|
3
|
Yes
|
No
|
Normal
|
|
|
2
|
|
|
Distal
|
|
|
1.5
|
|
|
|
Labioscrotal
|
Labioscrotal
|
1
|
|
|
Mid
|
Inguinal
|
Inguinal
|
0.5
|
|
|
|
Abdominal
|
Abdominal
|
0
|
No
|
Yes
|
Proximal
|
Absent
|
Absent
|
An EMS score <11 out of 12 needs further clinical investigation for DSD in 46,XY.
Table 7. External Genital Score (EGS)
|
External genital score (EGS)
|
Labio-scrotal fusion
|
Genital tubercle size (mm)
|
Urethral meatus
|
Right gonad
|
Left gonad
|
3
|
Fused
|
≥31
|
Typical male
|
|
|
2.5
|
|
26-30
|
Coronal glandular
|
|
|
2
|
|
|
Penile
|
|
|
1.5
|
Posterior fusion
|
21-25
|
Peno-scrotal
|
Labio-scrotal
|
Labio-scrotal
|
1
|
|
10-20
|
Scrotal
|
Inguino-scrotal
|
Inguino-scrotal
|
0.5
|
|
|
Perineal
|
Inguinal
|
Inguinal
|
0
|
Unfused
|
≤ 10
|
Typical female
|
Impalpable
|
Impalpable
|
Median EGS (10th to 90th centile) in males < 28 weeks gestation is 10 (8.6-11.5); in males 28-32 weeks 11.5 (9.2-12); in males 33-36 weeks 11.5 (10.5-12) and in full-term males 12 (10.5-12). In all female babies, EGS is 0 (0-0).
Table 8. Reference Ranges for Anogenital Distance and Anogenital Index in Term Neonates
|
|
5th
|
10th
|
25th
|
50th
|
75th
|
90th
|
95th
|
Males
|
|
|
|
|
|
|
|
AGD (mm)
|
18.47
|
19.44
|
21.00
|
22.68
|
25.20
|
27.80
|
29.08
|
AGI (mm/kg)
|
5.59
|
5.83
|
6.30
|
6.93
|
7.62
|
8.22
|
8.57
|
Females
|
|
|
|
|
|
|
|
AGD (mm)
|
9.40
|
9.85
|
10.55
|
11.65
|
12.60
|
13.45
|
14.10
|
AGI (mm/kg)
|
3.04
|
3.15
|
3.38
|
3.59
|
3.80
|
4.00
|
4.19
|
Anogenital index (AGI): the body weight standardized index of AGD [AGI=AGD/weight (mm/kg)] and found that using AGI as parameter has a better correlation with age.
Basic Auxiliary Investigations in the First Days
In the first few days of life, basic investigations are essential to evaluate adrenal and gonadal development and function in otherwise healthy newborns with ambiguous genitalia. Some key aspects to address include serum electrolytes and glucose levels. They should be monitored daily as cortisol deficiency can manifest as hypoglycemia in newborns affected by CAH. Body weight and feeding characteristics should also be monitored as excessive weight loss may indicate dehydration and electrolyte disturbances.
KARYOTYPE
Besides careful clinical evaluation, first-line investigation of an individual with DSD involves confirming the chromosomal sex using quantitative fluorescence polymerase chain reaction (QF-PCR) and karyotype (22). QF-PCR detects a series of markers on the sex chromosomes and has a turn-around time of about 1–2 days. This technique has largely replaced fluorescence in situ hybridization (FISH) methods. The analysis of karyotype is a cytogenetic technique (involving G-banding) and is crucial for the initial classification of a newborn with ambiguous genitalia (Table 3). Some centers have replaced karyotyping by array-comparative genomic hybridization (aCGH) or SNP array, with faster turn-around times of 5–10 days. But these techniques will not detect structural chromosomal rearrangements and may be less effective at detecting sex chromosome mosaicism.
HORMONE STUDIES
Detailed hormone studies may be indicated including serum gonadotropins (LH, FSH), androgens and androgen precursors (17-hydroxypregnenolone, 17-hydroxyprogesterone, androstenedione, testosterone, dihydrotestosterone), adrenal steroids and hormones (cortisol, aldosterone, and their precursors; ACTH, renin), and anti-Müllerian hormone (AMH) (1, 24).
Care must be taken when interpreting the results in premature babies, in whom these studies may need to be repeated at a later age and special normative value may apply. Even in term babies the timepoint and the clinical context (mode of delivery, drug effects, actual stress level etc.) need to be considered when evaluating hormonal data immediately after birth. Hormonal data change massively during delivery (e.g., surge) and within days thereafter. Additionally, it's essential to be aware that normative values for hormone levels can vary depending on the laboratory and the methods used for testing. The interpretation of hormonal data should be done by experienced healthcare providers and, if necessary, in consultation with a specialist in DSD.
IMAGING STUDIES
Ultrasound imaging of the abdominal-pelvic organs is a valuable diagnostic tool in the evaluation of newborns with ambiguous genitalia. It provides important insights into the internal reproductive and urinary structures, helping to determine the presence and characteristics of gonads, Müllerian structures (e.g., the uterus), and any associated abnormalities of the urinary tract. It can be performed at the first visit of a child with ambiguous genitalia. Of course, this investigation is not easy and may only be performed by an experienced ultra-sonographer to reveal meaningful results. Ultrasound can also detect abnormalities of the urinary tract (kidney, ureters, bladder) that sometimes occur in conjunction with genital ambiguity. Interestingly, data show that a child with a uterus and a female internal system that presents with ambiguous genitalia without gonads in the genital folds and has a 46,XX karyotype, will have in 80% of cases a diagnosis of a 46,XX CAH (most likely 21-hydroxylase deficiency). The information needed to come to this conclusion in a newborn with ambiguous genitalia can be available within 48 hours in institutions with an experienced DSD team.
In a second step, usually after months or years, additional imaging studies with MRI can be helpful in identifying both the type and extent of internal sex organ development in more details. An MRI may reveal further details on Müllerian structures (uterus remanent, fallopian tubes, upper portion of the vagina) than an ultrasound and can also help for localizing abdominal gonads. MRI may also be used for surveillance of the gonad at risk for cancer.
A genitogram had been used in the past (often early) to visualize the urinary tract, and to determine its position in relation to the vagina or vagino-utricular pouch but is no longer performed in most institutions.
However, in follow-up, cystoscopy and vaginoscopy may be used. These investigations are invasive procedures requiring general anesthesia, and therefore need to have a clear indication. Often, they are performed after complications such as urinary tract infections, to better understand the anatomy and its consequences, and in preparation for a surgical procedure (112).
Overall, the choice of imaging studies and procedures should be guided by the individual's clinical presentation, diagnosis, and the specific clinical questions that need to be addressed. In many cases, a multi-disciplinary DSD team collaborates to determine the most appropriate imaging approach to provide a comprehensive evaluation and guide treatment decisions.
GENETIC STUDIES
The rapid advancement of genetic technologies, particularly massive parallel sequencing methods like DSD panels, whole exome sequencing (WES), and whole genome sequencing (WGS), has significantly expanded our understanding of the genes involved in sex development. As a result, genetic workup has become an integral part of the recommended routine evaluation for individuals with DSD and thus ambiguous genitalia at birth (8, 22, 24, 113, 114).
While making a precise genetic diagnosis is still a challenge in some cases, the use of advanced genetic techniques has improved the diagnostic yield. Currently, a clear molecular-level diagnosis can be established in approximately half of individuals with 46,XY DSD who undergo genetic workup. However, as genetic technologies continue to evolve, and our understanding of sex development genes expands, it is likely that more cases, including those involving individuals with ambiguous genitalia at birth, will receive accurate and valid diagnoses. Therefore, re-review of genetically unsolved cases by a DSD team and genetic re-analysis by DSD-specialized teams is recommended to achieve a final diagnosis in as many as possible.
In summary, the integration of genetic workup, in combination with ongoing advancements in technology and genetic knowledge, is expected to enhance our ability to provide individuals with DSD, including those with ambiguous genitalia at birth, with more precise and comprehensive diagnoses in the future. This progress will lead to better-informed clinical decisions and management strategies for newborns with ambiguous genitalia.
DIFFERENTIAL DIAGNOSIS
Generally, the result from the karyotype plays a major role in the differential diagnosis and workup of DSD and thus ambiguous genitalia (Table 3). In most cases, the chromosomes in newborns with ambiguous genitalia will be either 46,XX or 46,XY. In rare instances, chromosomal aberration such as 45,X/46,XY or 46,XX/46,XY mosaicism may be the underlying cause explaining the phenotype. Additionally, measurements of specific hormones, such as serum luteinizing hormone (LH), follicle-stimulating hormone (FSH), sex steroids, AMH, inhibin B at birth and at follow-up, in the so-called mini-puberty of infancy period, can provide valuable information for the differential diagnosis of DSD and ambiguous genitalia.
In the following we provide two flowcharts and give some general information for the step-by-step workup of newborns with ambiguous genitalia and either 46,XX or 46,XY chromosomes.
46, XX DSD
A 46, XX karyotype in a newborn with ambiguous genitalia indicates that the child is a genetic female who was exposed to excessive amounts of androgens during fetal life. A flowchart for differential diagnosis is given in Figure 8.
Marked elevation of plasma 17-hydroxypregnenolone, 17-hydroxyprogesterone, 21-deoxycortisol and androstenedione, along with male-typical levels of testosterone, are characteristic of 21-hydroxylase deficiency (CYP21A2). High values of corticosterone and 11-deoxycortisol, along with elevated androgens, indicate 11β-hydroxylase deficiency (biallelic mutations in CYP11B1). In 3beta-HSD deficiency (biallelic mutations in HSD3B2), calculation of ratios of delta-5 (pregnenolone, 17OH-pregnenolone, DHEA) over delta-4 (progesterone, 17OH-progesterone, androstenedione) steroid metabolites (see Figure 4) will point towards the genetic defect.
Overall, comprehensive steroid profiling from blood or urine using chromatographic, mass spectrometric methods allow the diagnosis of most steroidogenic defects causing androgen excess CAH in 46,XX newborns (115-117) (see also Table 5). In addition, they have led to the description of novel alternate pathways to produce active androgens in the intermediate metabolism through the backdoor or 11-oxygenated pathways, especially in disorders such as 21-hydroxylase or POR deficiencies, thereby enhancing our understanding and diagnostic possibilities (115, 117, 118)(Figure 4). However, these methods are not (yet) widely available, and the interpretation of these data is not trivial. In addition, instruments and methods vary from lab to lab. Thus, method validation is tedious and comparison between labs difficult so far (119-121).
When excess maternal androgen production is the underlying cause for masculinization of a female fetus, the source of these steroids is eliminated postnatally. Thus, the various steroids studied in affected newborns will be in the female-typical range despite masculinization of the external genitalia. Then, detailed examination of the mother may provide the explanation.
Likewise, in cases of mild POR deficiency or aromatase deficiency, in which the feto-placental unit was not able to metabolize the fetal androgens and led to ambiguous genitalia, the problem will not (always) be visible in the newborn’s steroid metabolome, and the diagnosis may only be made by genetic testing. If not, only in later life, when aromatase activity is needed for estrogen production in puberty, or when ovarian cysts hint an underlying problem, the diagnosis may be made (Figure 8).
In individuals with ovo-testicular DSD and 46,XX chromosomes, masculinization arises from androgens secreted by the testicular portion of the differentiated gonads. Androgen production is similar to that produced by testes in unaffected males except that the amount is usually smaller. The degree of masculinization of the genitalia is thus linked to the amount of functioning testicular tissue, which can be determined by a serum AMH measurement (Table 9) (122).
Very low values of AMH and female-typical values for LH/FSH (Tables 9 and 10) are expected in 46,XX newborns with masculinized/virilized genitalia that is attributed to CAH or excess maternal androgen production during gestation (122, 123). AMH is higher in newborns with ovo-testicular DSD, due to Sertoli cell development in the testicular portion of their gonads where AMH is mainly produced.
On occasion, translocation of the pseudo-autosomal part of the Y chromosome along with a mutated SRY gene to an X chromosome occurs. The result is partial masculinization of the genitalia in a 46,XX newborn. With maturity, the phenotype of affected individuals closely resembles that of boys and men with Klinefelter syndrome.
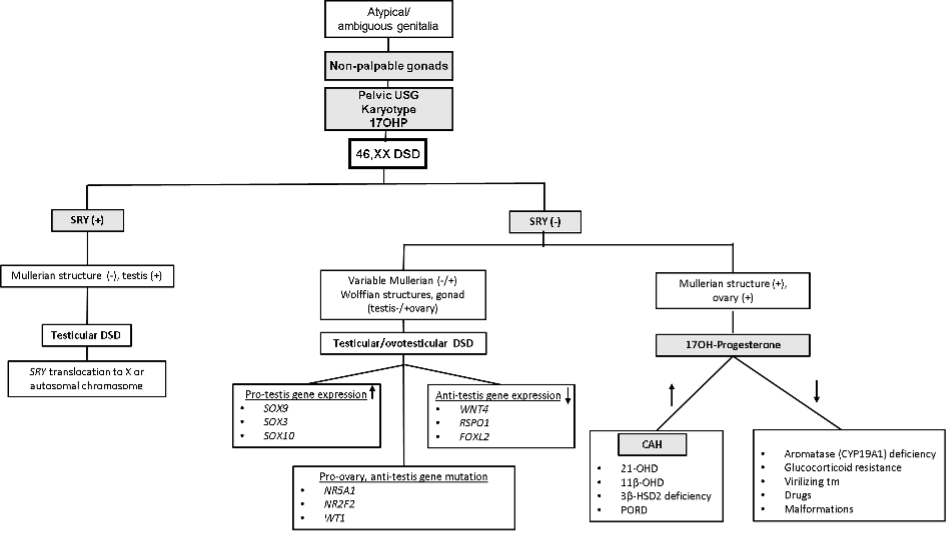
Figure 8. 46,XX DSD decision tree diagram.
Abbreviations: USG: Ultrasound; 17OHP: 17OH-Progesterone; DSD: Differences in sex development; CAH: Congenital adrenal hyperplasia; 21OHD: 21α-Hydroxylase deficiency; 11β-OHD: 11β-Hydroxylase deficiency; 3β-HSD2: 3β-Hydroxysteroid dehydrogenase type 2; PORD: P450 oxidoreductase deficiency.
Table 9. Normal Values for AMH (ng/mL)* in Fetuses, Newborns and Infants Under 1 Year (122)
|
|
Female
|
Male
|
|
n
|
Median
|
5-95th percentile
|
n
|
Median
|
5-95th percentile
|
Fetal blood
|
25
|
<0.4
|
<0.4-0.5
|
23
|
44.4
|
13.4-86.2
|
Cord blood
|
53
|
<0.4
|
<0.4-1.1
|
48
|
30.7
|
7.4-610.7
|
1-30 days
|
70
|
<0.4
|
<0.4-1.3
|
73
|
57.2
|
23.8-124.0
|
31-120 days
|
31
|
0.8
|
<0.4-6.4
|
39
|
88.4
|
46.8-173.0
|
4-12 months
|
13
|
1.0
|
<0.4-2.7
|
53
|
124.7
|
67.4-197.0
|
* AMH/MIS enzyme immunoassay kit cat 2368, Immunotech-Beckman Coulter, France
Table 10. LH and FSH Values (means ± SD) From Female and Male Newborns. Modified from (123)
|
|
|
Female
|
Male
|
Age (days)
|
Hormone
|
n
|
Median
|
Mean±SD
|
n
|
Median
|
Mean±SD
|
1-5
|
FSH (IU/L)
|
31
|
1.80
|
2.00 ± 1.37
|
30
|
0.85
|
0.96 ± 0.60
|
LH (IU/L)
|
0.20
|
0.48 ± 0.66
|
0.20
|
0.39 ± 0.48
|
6-10
|
FSH (IU/L)
|
17
|
1.40
|
2.44 ± 2.52
|
15
|
1.40
|
2.91 ± 4.38
|
LH (IU/L)
|
0.30
|
0.45 ± 0.33
|
0.30
|
0.45 ± 0.33
|
11-15
|
FSH (IU/L)
|
8
|
8.95
|
8.16 ± 4.27
|
17
|
3.00
|
3.71 ± 2.69
|
LH (IU/L)
|
1.60
|
1.58 ± 1.28
|
2.90
|
3.55 ± 2.84
|
16-20
|
FSH (IU/L)
|
6
|
1.90
|
1.62 ± 1.05
|
14
|
2.15
|
2.63 ± 1.45
|
LH (IU/L)
|
0.35
|
1.03 ± 1.39
|
3.65
|
4.13 ± 2.76
|
21-25
|
FSH (IU/L)
|
3
|
3.90
|
7.07 ± 5.92
|
7
|
2.10
|
2.50 ± 1.51
|
LH (IU/L)
|
0.50
|
0.46 ± 0.25
|
2.70
|
2.86 ± 1.51
|
26-28
|
FSH (IU/L)
|
8
|
6.15
|
9.74 ± 9.89
|
8
|
2.40
|
2.25 ± 0.81
|
LH (IU/L)
|
2.80
|
2.75 ± 2.39
|
1.40
|
2.22 ± 2.37
|
Abbreviations: LH, luteinizing hormone; FSH, follicle-stimulating hormone.
46, XY DSD
A 46, XY karyotype reveals that one is dealing with a genetic male who was under-masculinized during fetal development. A flowchart for differential diagnosis is given in Figure 9.
Laboratory findings of normal or elevated testosterone and DHT with normal AMH (Figure 9) indicate a diagnosis of AIS. If testosterone levels are normal but DHT levels are low, a diagnosis of steroid 5α-reductase deficiency can be made. Low levels of testosterone and DHT, along with marked elevation of some androgen precursors, indicate a deficiency of one of the enzymes required for androgen biosynthesis (Figure 9). For example, if the elevated precursors include androstenedione and 17-hydroxyprogesterone, then the defective enzyme is 17-ketosteroid reductase (HSD17B3). In all cases of testosterone biosynthetic defects, AMH levels are similar to those observed in healthy 46,XY male infants with male-typical external genitalia (Figure 9). With early defects of steroidogenesis that affect both adrenal and gonadal function, it is though important to evaluate adrenal function, as it might be impaired with deficiencies of STAR, CYP11A1, HSD3B2, CYP17A1 and POR (Table 5, Figure 9).
Finally, when all androgens and their precursors are below normal levels, one is dealing with gonadal dysgenesis or 46,XY ovo-testicular DSD. In these cases, AMH values should also be low. In contrast, for babies affected by Leydig cell hypoplasia, androgens and their precursors are low, while AMH values should be in the normal male range.
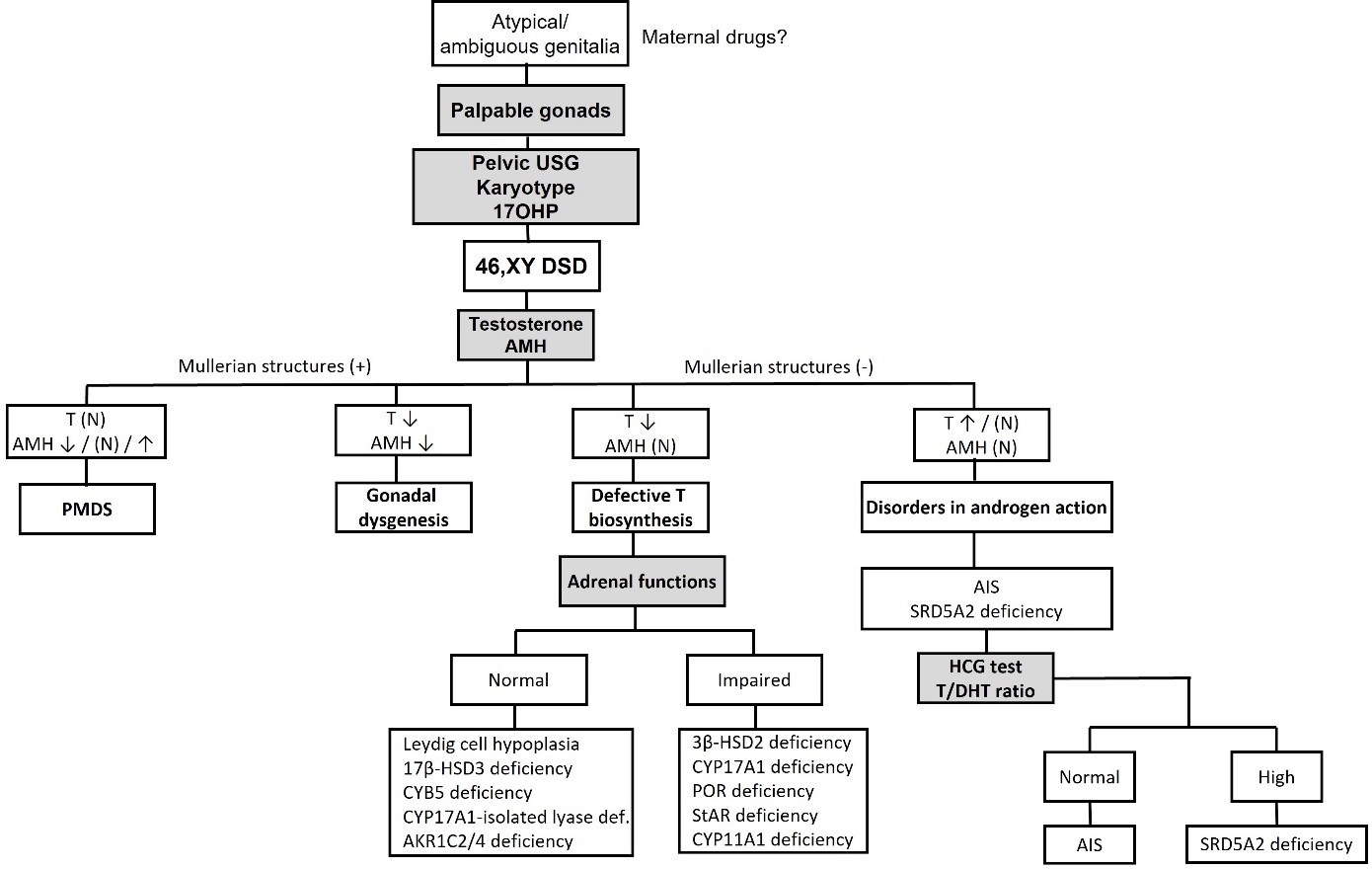
Figure 9. 46,XY DSD decision tree diagram.
Abbreviations: USG: Ultrasound; 17OHP: 17OH-Progesterone; DSD: Differences in sex development; AMH: Anti-Müllerian hormone; T: Testosterone; N: Normal; PMDS: Persistent Müllerian duct syndrome; AIS: Androgen insensitivity syndrome; SRD5A2: 5α-reductase A2; HCG: Human chorionic gonadotropin; DHT: Dihydrotestosterone; 3β-HSD2: 3β-Hydroxysteroid dehydrogenase type 2; CYP17A1: 17α-Hydroxylase/17-20 lyase; POR: P450 oxidoreductase; StAR: Steroidogenic acute regulatory protein; CYP11A1; Cholesterol side-chain cleavage enzyme; 17β-HSD3: 17β-Hydroxysteroid dehydrogenase type 3; AKR1C2/4: Aldo-keto reductase family 1 member C2/4; CYB5: Cytochrome b5.
Testing Early Postnatal Gonadal Function and Mini-Puberty
In cases of ambiguous genitalia where a diagnosis may not be reached through first line investigations, and where a gonadal malfunction is suspected, functional testing and/or re-analysis of gonadal function at the timepoint of mini-puberty may be helpful, especially also with respect to having information when making considerations towards sex of registration (e.g., in utero effect of sex hormones on the brain) and prediction of later pubertal development and fertility potential.
Mini-puberty is the time window at day 30-100 after birth, when the hypothalamic-pituitary-gonadal (HPG) axis is re-activated for a short time frame in infancy, before going to inactivity until puberty. Little is known about the purpose of mini-puberty, but as the HPG axis is active and stimulates the gonads, it is a time window of opportunity to assess gonadal function without the necessity to stimulate with human chorionic gonadotrophin (hCG).
As a principle, in mini-puberty, the typical ovaries of a 46,XX newborn show little functional activity, while the typical testes of a 46,XY newborn are quite (re)active in testosterone (T), DHT, AMH and inhibin B production. While androgens are produced in the Leydig cells of the testes, AMH and inhibin B originate from the Sertoli cells. Table 11 gives an overview of the hormonal values observed during normal mini-puberty in healthy 46,XX and 46,XY infants (124).
Table 11. Evaluation of Reproductive Hormones During Mini-Puberty in Infants. Normative Values of Different Serum Hormones in 46,XX Female and 46,XY Male Infants. Modified from (124)
|
|
|
Females
|
Males
|
|
Post-natal time (months)
|
n
|
GMean
|
2.5-97.5th percentile
|
n
|
GMean
|
2.5-97.5th percentile
|
LH (IU/L)
|
2-3.5
|
432
|
<LOD
|
<LOD-0.98
|
581
|
1.71
|
0.62-4.08
|
3.5-5
|
110
|
<LOD
|
<LOD-1.25
|
166
|
1.40
|
0.54-3.32
|
FSH (IU/L)
|
2-3.5
|
435
|
3.98
|
1.23-17.4
|
578
|
1.19
|
0.41- 3.02
|
3.5-5
|
111
|
3.93
|
1.30-17.7
|
165
|
1.11
|
0.42-2.68
|
Testosterone (RIA,nmol/L)
|
2-3.5
|
74
|
<LOD
|
<LOD-0.40
|
592
|
3.04
|
0.69-7.60
|
3.5-5
|
14
|
<LOD
|
<LOD-NA
|
168
|
1.97
|
<LOD-7.01
|
Testosterone
(LC-MS/MS, nmol/L)
|
2-3.5
|
165
|
<LOD
|
<LOD-0.21
|
251
|
4.75
|
1.35- 11.3
|
3.5-5
|
125
|
<LOD
|
<LOD-0.17
|
175
|
2.25
|
0.32- 9.65
|
Estradiol (pmol/L)
|
2-3.5
|
455
|
29
|
<LOD-79
|
571
|
<LOD
|
<LOD-47
|
3.5-5
|
113
|
31
|
<LOD-98
|
160
|
<LOD
|
<LOD-49
|
SHBG (nmol/L)
|
2-3.5
|
427
|
133
|
67-264
|
579
|
135
|
66-268
|
3.5-5
|
110
|
141
|
72-282
|
162
|
143
|
71-262
|
Inhibin B (pg/mL)
|
2-3.5
|
423
|
62
|
<LOD-184
|
571
|
379
|
229-631
|
3.5-5
|
106
|
67
|
<LOD-174
|
158
|
379
|
222-662
|
AMH (pmol/L)
|
2-3.5
|
339
|
11
|
<LOD-49
|
48
|
1013
|
425- 1810
|
3.5-5
|
67
|
15
|
2-46
|
12
|
1183
|
797-NA
|
Abbreviations: LOD, Limit of detection; GMean, geometric mean; LH, luteinizing hormone; FSH, follicle-stimulating hormone; T, testosterone; RIA, radioimmunoassay; SHBG, sex-hormone binding globulin; AMH, anti-Müllerian hormone; LC-MS/MS, liquid chromatography-tandem mass spectrometry; NA, not available.
HCG Testing of Gonadal Function in a DSD Newborn
The human chorionic gonadotrophin (hCG) test can assess whether in a newborn with ambiguous genitalia, functioning Leydig cells are present. The test may therefore be useful for diagnosing testosterone biosynthesis defects such as HSD17B3 or SRD5A2 deficiency (Table 12). Several protocols for the hCG test exist, but all baseline serum samples are taken for T, DHT and precursors. Then, one to three intramuscular injections of high dose hCG (500-1500 IU) are given at 24 hours interval, and finally repeat stimulated samples are taken for androgen measurements after 72 or 24 hours, respectively, after the last injection. The following Table 12 shows how results of the test may be interpreted in various DSD conditions that may lead to ambiguous genitalia (125, 126).
Table 12. Interpretation of hCG Stimulation Test Results (125, 126)
|
Indication
|
Consideration
|
Abnormal result
|
Testosterone biosynthesis defects
|
T before and after hCG administration
|
T increase < 100-150 ng/dL (3.5-5 nmol/L)
|
5α-Reductase deficiency
|
T/DHT ratio after hCG administration
|
≥ 8.5 for minipuberty,
≥ 10 for prepuberty,
≥ 17 for puberty,
> 20 in genetically proven cases using LC-MS/MS
|
17β-HSD3 deficiency
|
T/A4 ratio after hCG administration
|
< 0.8
|
AIS
|
T, SHBG before and after hCG administration
|
T increases but SHBG does not change significantly
|
Abbreviations: T, testosterone; DHT, dihydrotestosterone; HCG, human chorionic gonadotropin; A4, androstenedione; SHBG, sex-hormone binding globulin; AMH, anti-Müllerian hormone; LC-MS/MS, liquid chromatography-tandem mass spectrometry; 17β-HSD3 deficiency, 17β-hydroxysteroid dehydrogenase type 3 deficiency; AIS, androgen insensitivity syndrome
RISK OF MALIGNANCY OF THE DSD GONAD
Individuals with certain disorders of sex development (DSD), especially those involving Y chromosome material, have an increased risk of developing gonadal tumors. These tumors are mainly gonadal germ cell tumors (GGCTs), which occur in about 15 % of all DSD cases, with the risk varying depending on the age and specific underlying diagnosis. Gonadal stromal tumors and smooth muscle leiomyomas/hamartomas are less common and are found in only about 0.9% of DSD cases (127). Risk factors for development of gonadal malignancy in DSD are shown in Figure 10. Several factors contribute to the increased risk of gonadal malignancy in individuals with DSD, including genetic factors, the presence of Y chromosome material, and the type of DSD. Therefore, ongoing monitoring and appropriate management are essential to minimize this risk. While gonadectomy (surgical removal of the gonads) is not recommended for every case of 46,XY DSD, it's crucial to consider the risk of malignant tumors in the decision-making process (1, 69). The consensus statement on the management of DSD provides guidelines and recommendations regarding the timing of gonadectomy for specific patients. These recommendations aim to balance the potential benefits of gonadectomy with the associated risks and individual patient needs.
In individuals with DSDs, germ cells typically undergo a process of apoptosis (programmed cell death) as the body grows and develops. This process eliminates germ cells that are not needed for reproduction. However, in some cases, certain germ cells may not undergo the normal maturation process and remain in an immature state. These immature germ cells can continue to express embryonic markers such as octamer-binding transcription factor 3/4 (OCT3/4) encoded by POU5F1; stem cell factor (SCF), also known as c-KIT ligand (encoded by KITLG); and placental alkaline phosphatase (PLAP). These cells are susceptible to developing into cancerous lesions, starting as pre-invasive lesions (gonado-blastoma) or as precursors to invasive GGCT (dysgerminoma) (Figure 10).
DSD patients who have Y chromosome material in their gonadal karyotype are at risk of GGCTs regardless of the degree of testicular differentiation. Y chromosome material associated with gonadal tumors is GBY locus which is around the centromere of the Y chromosome and includes the gene for ¨testis-specific protein on the Y chromosome¨ (TSPY, located in Yp11.2). Other potentially related genes are SRY and DYZ3.
The risk of developing gonadal tumors in gonadal dysgenesis may also vary according to the underlying molecular etiology. Patients with genetic defects that lead to early blockage of gonadal development such as mutation or deletions of SRY or WT1 have a higher risk. The risk of developing gonadal tumors ranges from 40% to 60% in gonadal dysgenesis due to WT1 gene mutations (Frasier syndrome and Denys-Drash syndrome). Early bilateral gonadectomy is therefore recommended. Similarly, early bilateral gonadectomy is recommended in patients with complete or partial gonadal dysgenesis due to SRY gene mutations due to the 20% to 52.5% risk of gonadal tumor development. Gonadal dysgenesis due to MAP3K1 gene mutations is another indication for early gonadectomy (127). Gonadal tumor risk is also present, though somewhat lower in gonadal dysgenesis due to 45,X0/46,XY mosaicism. The exact time of malignancy development and the prevalence of tumors in other molecular etiologies require further research for a comprehensive understanding.
Histologically the risk of gonadal tumor development increases with gonadal immaturity. Fully developed gonads such as the testes, ovary or ovotestis have lower risk of malignancy than streak or dysgenetic testes (Figure 10). In ovo-testicular DSD, the risk of germ cell tumors is low (3%) (1). Newborns with PAIS are at high risk (50%) for developing gonadal tumors, and bilateral orchiopexy or gonadectomy is recommended at the time of diagnosis, if the testes are located in the abdomen (1, 128).
The risk of developing germ cell malignancy in newborns with CYP11A1, HSD3B2 or CYP17A1 deficiency is unknown. Newborns with 17-ketosteroid reductase deficiency have a fairly low risk for malignancy (1). Tumor risk in newborns with Leydig cell hypoplasia is unclear, and thus the recommendation for gonadectomy is not well established (1, 25, 129, 130).
Overall, it remains challenging to predict the personal risk of gonadal neoplasia (mostly GGCT) for a DSD individual, because it depends on many other factors besides genetics. Stimulating gonadotropins and gonadal hormones during mini-puberty and after puberty onset are also regarded as risk factors increasing gonadal tumor risk in patients with DSD. Similarly, gonads located intra-abdominal carry a higher tumor risk. The management of gonadal tumor risk in individuals with DSD is a complex issue that requires careful consideration and a personalized approach, taking into account the specific DSD subtype, genetic factors, and patient preferences. Regular monitoring and discussions with a healthcare team experienced in DSD management are essential to make informed decisions about gonadectomy. If gonads are located in the scrotum, then periodic examination of the testes may be advised as an alternative to gonadectomy, while an intra-abdominal gonad at risk may only be monitored by imaging methods such as ultrasound or MRI.
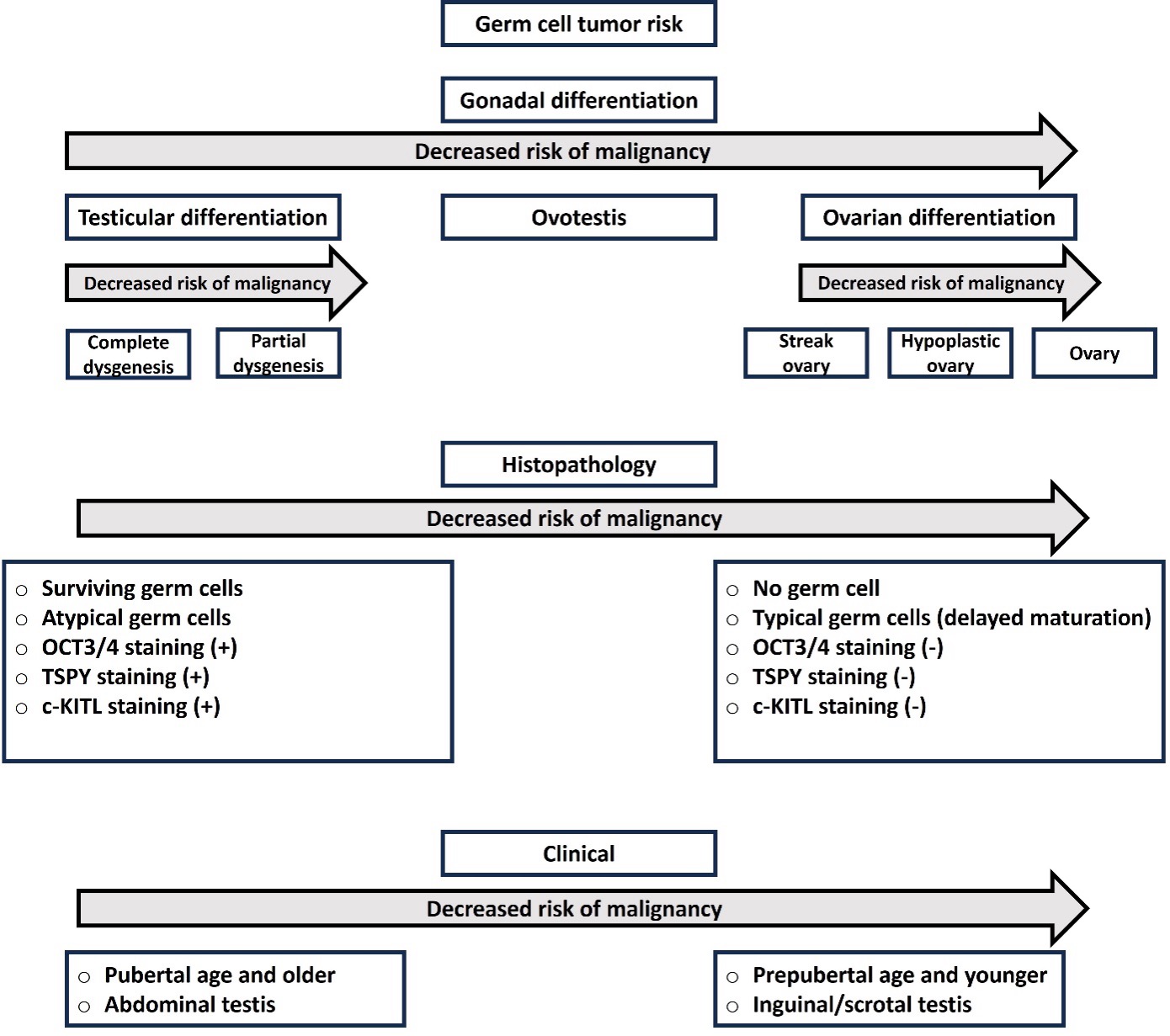
Figure 10. Risk of malignancy development in the DSD gonad.
Abbreviations: (OCT3/4); Octamer-binding transcription factor 3/4, TSPY; testis-specific protein on the Y chromosome, c-KITL; c-KIT ligand also called stem cell factor (SCF).
SEX REGISTRATION OF A NEWBORN
Assigning or registering the sex of a newborn with atypical external genitalia is a complex and important decision that requires careful consideration, consultation, and a patient-centered approach. The parents and DSD network team should not rush but take the time needed to come to a consensus in the best interest of the child in its environment. Not knowing whether the newborn is a girl or boy causes mostly a lot of distress and one is tempted to call it an emergency, but if there are no medical reasons to make it an emergency (like e.g., suspected adrenal insufficiency or additional organ anomalies), it is important to declare it accordingly and inform that the newborn is not at risk and actually does not care whether it is a male or female or intersex person at this moment. Thus, the stress of ambiguity of the newborn is actually at this moment “only” an issue of the parents and the care team who mostly wish to know ASAP whether the child can be assigned/registered a “correct” sex that might also fit later in life.
To consider sex registration, not only is it important to take into account the underlying cause and knowledge on the possible outcome of the specific, often unique condition, but also the family, cultural, and societal preconditions and aspects. Most societies have a binary sex registration obligation by legislation, but some societies have more recently introduced a third sex category besides male and female. Although this seems to make things easier, it has been discussed that such categorization in a third or other or unclassified group might also stigmatize a person in a society that is mostly binary. Thus, in real life practice, there is rumor that a third sex category is rarely chosen for a newborn with ambiguous genitalia by the parents and the DSD team. Overall, a personalized approach for every newborn with ambiguous genitalia involving the parents and the DSD network team is recommended following the shared-decision-making process (12, 13).
Most important is that the team around the newborn with ambiguous genitalia works together in the best interest of the child with the latest knowledge of the literature and expertise when taking the decision of sex registration, and still remains humble and open minded for the child’s future that may follow a different path leading to sex reassignment later in life. Therefore, continuous support of the family and the child by a DSD psychologist is extremely helpful to recognize early signs of gender dysphoria, inform the child about the condition in an age-appropriate manner and follow its will, if case sex reassignment is desired.
Generally, people believe that the sex chromosomes indicate a person’s “true sex” and laws exist supporting this idea, to the detriment of some individuals affected by DSD (131). Scientifically speaking, it is clear that the majority of genes on the X chromosome do not influence sex development and differentiation, although the AR gene is necessary for phenotypic masculinization. Concerning the Y chromosome, only the SRY gene contributes to testicular formation. In fact, most of the genes required for sex development and differentiation are found on the autosomes (1). Therefore, chromosomes do not dictate the sex of rearing in newborns affected by a DSD and are only one piece of a big puzzle (Figure 3).
Although there is no overall recommendation for sex registration at birth for a child with ambiguous genitalia, some helpful information is available from larger patients’ cohorts with a specific DSD diagnosis in the literature.
Newborns presenting with 46,XY chromosomes and female external genitalia at birth (thus actually not with ambiguous genitalia) due to complete androgen insensitivity syndromes (CAIS), complete gonadal dysgenesis (Swyer syndrome), or other complete loss of testosterone biosynthesis live successfully when assigned a female sex of rearing. Female assignment in such cases is widely accepted by patients throughout their lives despite challenges that they may experience regarding sexual dysfunction and infertility (1, 132-134).
For newborns with ambiguous genitalia affected by partial gonadal dysgenesis, no general recommendation can be made. Fewer procedures were historically required for surgical feminization compared to surgical masculinization of the genitalia. However, the functional outcome in individuals who received feminizing or masculinizing procedures is less than optimal (135, 136). Additionally, rates of satisfaction with sex of rearing are similar for affected individuals whether raised male or female (1, 20, 137). Thus, the decision should not be made on the basis of “surgical possibilities”, and overall, such surgical procedures should be executed with reservation. Clearly, further studies are needed to elucidate why some individuals with partial gonadal dysgenesis experience gender dysphoria, while others do not.
Likewise, no general recommendation can be made for patients with ambiguous genitalia due to a partial androgen biosynthesis defect, (e.g., partial 3βHSD2, CYP17A1, POR, StAR, CYP11A1 deficiencies). But some studies suggest that the degree of masculinization of the external genitalia may reflect the degree of virilization of the brain and thus the gender preference of this person (138). This might be true for 46,XY newborns with ambiguous genitalia that are manifesting with severe hypospadias, but not without exceptions. For patients with variants in the SRD5A2 or HSD17B3genes recognized with ambiguous genitalia at birth (or even missed because manifesting with a female-typical external genitalia), there is quite a strong body of data recommending male sex registration at birth as this is the preferred sex of these persons when asked in later life (139, 140). Normal virilization at puberty, coupled with intact fertility potential, are strong factors for this recommendation at birth. If registered as female at birth and diagnosed later, gender dysphoria and desire of sex reassignment later in life is very likely.
Similar to partial gonadal dysgenesis, consensus regarding an optimal sex of registration for newborns affected by PAIS does not exist. Although earlier data showed that fewer procedures were usually required for surgical feminization, compared to surgical masculinization, in a person with ambiguous external genitalia in the past, when larger series of genital surgery were analyzed, it is unclear if the functional outcome was better among newborns who received feminizing procedures compared to those who received masculinizing procedures. Rates of satisfaction with sex of rearing are similar for individuals with PAIS raised female or male (132, 141). While reports exist of impaired sexual function in people with PAIS raised male, it is highly suspected that sexual functional outcomes in affected people raised female are similarly poor (137). Reports of sexual satisfaction for people with PAIS who have not received genitoplasty in infancy, or at a later age, are limited. However, more information on outcome of newborns with ambiguous genitalia who had no genital surgery, at least not in infancy and childhood, will hopefully soon be added to the medical literature as current developments recommend abstaining from genital surgery.
By contrast, in 46,XX newborns with ambiguous genitalia due to CAH, it is generally recommended to register them a priori in the female sex, even if they are severely virilized (142-144). Masculinization due to 21-hydroxylase deficiency does not impair the development of the ovaries or the Müllerian ducts and thus fertility potential. Limited information is available about the medical and psychosexual outcome of 46,XX newborns affected by 21-hydroxylase deficiency and raised male (145, 146), although these patients do tend to have an increased risk of gender dysphoria compared to their female counterparts (147). Thus, a female sex of rearing is typically the decision for 46,XX newborns affected by 21-hydroxylase deficiency (145). Female sex of rearing in 46,XX newborns with ambiguous genitalia is also recommended for conditions resulting from maternal overexposure to androgens and placental aromatase deficiency.
Only recently has the understanding of, and reaction to, having a child with ambiguous genitalia received systematic study. For some parents and caregivers, feelings of isolation and concern over what the future may hold for their affected child in terms of stigmatization and sexual dysfunction are paramount (148). One potential approach to ameliorate this issue is to provide information and management options to the family early in pregnancy or as soon as the child is born. Guidance by a DSD network team is therefore extremely important.
Some research has been done to examine the effects of prenatal diagnosis and treatment. In females with 21-hydroxylase deficiency CAH, one of the most common causes of ambiguous genitalia, dexamethasone treatment of the pregnant mother has been reported to reduce virilization of an affected female fetus by 80-85% (149), but this approach is no longer offered or recommended in guidelines because of potential adverse effects of dexamethasone on the developing fetus. Before the discovery of methods to measure fetal DNA in maternal blood, which enabled specific diagnosis of sex and whether the fetus inherited and was affected by the mutation, controversies existed because 7 unaffected fetuses would be treated unnecessarily (1:4 chance of inherited mutation and 1:2 chance of male vs female), So, on the one hand 7 unaffected fetuses and the mother would be exposed to an unnecessary drug, while on the other hand, even in affected fetuses long-term outcome showed adverse effects on neurocognitive parameters (150). Today, noninvasive prenatal diagnosis using massive parallel sequencing of cell-free fetal DNA can be used to determine a specific diagnosis, such as CAH, as early as 6 weeks of gestation (149, 151). Even preimplantation diagnostics is available (152). These techniques could be used to look at other autosomal dominant or X-linked conditions that cause ambiguous genitalia, especially when the fetus is known to be at risk (151). Further studies need to be done on this subject, but for families who already have an affected child or know that they are carriers, this may be an option that needs to be discussed with a DSD geneticist.
Patients for whom prenatal diagnosis and/or treatment is not an option, prognosis and management has improved significantly over time, but many open questions and controversies still exist. Our studies of parents of children with DSD reveal that the appearance of atypical genitalia can result in significant stress and maladaptive parenting strategies. For example, mothers of children with ambiguous genitalia experience greater stress if their child has not received “corrective” genital surgery (153) and some parents believe such surgeries eliminate stigmatization that may arise due to their child’s ambiguous genitalia However, as their children mature past infancy, some parents realize that their child’s DSD has not been ameliorated by genitoplasty, and concerns for their son or daughter resurface (148). To illustrate, among parents studied by our group, caregivers of adolescents with DSD experienced increased stress as their child matured despite the fact that many had received earlier surgery (154). Additionally, parents of children reared male reported the most perceived child vulnerability for their sons (155),and their reported levels of depression were directly associated with more atypical genital appearance for their sons (156). Overall, parents report that while early genitoplasty seems to “fix” some of their concerns for their child with DSD, this “fix” is not long-lasting. Instead, what needs to be emphasized to families is how to support their child who is different, but not damaged. Family centered, interdisciplinary care, with open and clear communication between patients, parents, and caregivers has been shown to be essential for optimal quality of life (157). In addition, networks of families who have the personal experience of parenting children with ambiguous genitalia can be an invaluable resource (158). Reservation towards early interventions needs to be considered,keeping in mind to preserve the personal right of the child to take his/her own decision towards body integrity. Any intervention that can be delayed without harming the newborn with ambiguous genitalia should be postponed until personal consent can be given. Finally, referring parents to support groups and introducing them to other caregivers of children born with ambiguous genitalia is extremely important to optimize parents’ understanding and acceptance of their child’s condition. With increased understanding and acceptance, optimal growth and development for children born with ambiguous genitalia may be obtained.
In conclusion, this Chapter give some insight into the current diagnostic evaluation and care of the newborn with ambiguous genitalia. We underscore the importance of a multidisciplinary and compassionate approach to the care of newborns with ambiguous genitalia, acknowledging the complex medical, psychological, and emotional aspects involved in such cases. We highlight the critical role of specialized teams and support in ensuring the well-being of both the child and his/her family.
REFERENCES
- Hughes IA, Houk C, Ahmed SF, Lee PA. Consensus statement on management of intersex disorders. Arch Dis Child. 2006;91(7):554-63.
- Halil H, Oguz SS. Establishment of normative data for stretched penile length in Turkish preterm and term newborns. Turk J Pediatr. 2017;59(3):269-73.
- Holmes NM, Miller WL, Baskin LS. Lack of defects in androgen production in children with hypospadias. J Clin Endocrinol Metab. 2004;89(6):2811-6.
- Alaei M, Rohani F, Norouzi E, Hematian Boroujeni N, Tafreshi RI, Salehiniya H, Soheilipour F. The Nomogram of Clitoral Length and Width in Iranian Term and Preterm Neonates. Front Endocrinol (Lausanne). 2020;11:297.
- Cools M, Nordenström A, Robeva R, Hall J, Westerveld P, Flück C, et al. Caring for individuals with a difference of sex development (DSD): a Consensus Statement. Nat Rev Endocrinol. 2018;14(7):415-29.
- Gorduza DB, Quigley CA, Caldamone AA, Mouriquand PDE. Surgery of Anomalies of Gonadal and Genital Development in the "Post-Truth Era". Urol Clin North Am. 2018;45(4):659-69.
- McElreavey K, Bashamboo A. Monogenic Forms of DSD: An Update. Horm Res Paediatr. 2023;96(2):144-68.
- O'Connell MA, Atlas G, Ayers K, Sinclair A. Establishing a Molecular Genetic Diagnosis in Children with Differences of Sex Development: A Clinical Approach. Horm Res Paediatr. 2023;96(2):128-43.
- Brown J, Warne G. Practical management of the intersex infant. J Pediatr Endocrinol Metab. 2005;18(1):3-23.
- Dayner JE, Lee PA, Houk CP. Medical treatment of intersex: parental perspectives. J Urol. 2004;172(4 Pt 2):1762-5; discussion 5.
- Lightfoot S, Carley M, Brinkman W, Gardner MD, Gruppen LD, Liang N, et al. Co-creating a suite of patient decision aids for parents of an infant or young child with differences of sex development: A methods roadmap. Front Urol. 2023;2.
- Légaré F, Witteman HO. Shared decision making: examining key elements and barriers to adoption into routine clinical practice. Health Aff (Millwood). 2013;32(2):276-84.
- Sandberg DE, Gardner M, Kopec K, Urbanski M, Callens N, Keegan CE, et al. Development of a decision support tool in pediatric Differences/Disorders of Sex Development. Semin Pediatr Surg. 2019;28(5):150838.
- Martinez de LaPiscina I, Fluck CE. Genetics of human sexual development and related disorders. Curr Opin Pediatr. 2021;33(6):556-63.
- Siiteri PK, Wilson JD. Testosterone formation and metabolism during male sexual differentiation in the human embryo. J Clin Endocrinol Metab. 1974;38(1):113-25.
- Wilson JD, Griffin JE, Russell DW. Steroid 5 alpha-reductase 2 deficiency. Endocr Rev. 1993;14(5):577-93.
- Güran T. Latest Insights on the Etiology and Management of Primary Adrenal Insufficiency in Children. J Clin Res Pediatr Endocrinol. 2017;9(Suppl 2):9-22.
- Ali SR, Lucas-Herald A, Bryce J, Ahmed SF. The Role of International Databases in Understanding the Aetiology and Consequences of Differences/Disorders of Sex Development. Int J Mol Sci. 2019;20(18).
- Wiesemann C, Ude-Koeller S, Sinnecker GH, Thyen U. Ethical principles and recommendations for the medical management of differences of sex development (DSD)/intersex in children and adolescents. Eur J Pediatr. 2010;169(6):671-9.
- Lee PA, Nordenström A, Houk CP, Ahmed SF, Auchus R, Baratz A, et al. Global Disorders of Sex Development Update since 2006: Perceptions, Approach and Care. Horm Res Paediatr. 2016;85(3):158-80.
- Krishnan S, Wisniewski AB. Ambiguous genitalia in newborns. Genetic Steroid Disorders. 2014:87-97.
- Audi L, Ahmed SF, Krone N, Cools M, McElreavey K, Holterhus PM, et al. GENETICS IN ENDOCRINOLOGY: Approaches to molecular genetic diagnosis in the management of differences/disorders of sex development (DSD): position paper of EU COST Action BM 1303 ‘DSDnet’. Eur J Endocrinol. 2018;179(4):R197-r206.
- Pyle LC, Nathanson KL. A practical guide for evaluating gonadal germ cell tumor predisposition in differences of sex development. Am J Med Genet C Semin Med Genet. 2017;175(2):304-14.
- Yatsenko SA, Witchel SF. Genetic approach to ambiguous genitalia and disorders of sex development: What clinicians need to know. Semin Perinatol. 2017;41(4):232-43.
- Zenteno JC, Canto P, Kofman-Alfaro S, Mendez JP. Evidence for genetic heterogeneity in male pseudohermaphroditism due to Leydig cell hypoplasia. J Clin Endocrinol Metab. 1999;84(10):3803-6.
- Ogata T, Matsuo N, Hiraoka N, Hata JI. X-linked lissencephaly with ambiguous genitalia: delineation of further case. Am J Med Genet. 2000;94(2):174-6.
- Reardon W, Gibbons RJ, Winter RM, Baraitser M. Male pseudohermaphroditism in sibs with the alpha-thalassemia/mental retardation (ATR-X) syndrome. Am J Med Genet. 1995;55(3):285-7.
- Biason-Lauber A, Konrad D, Meyer M, DeBeaufort C, Schoenle EJ. Ovaries and female phenotype in a girl with 46,XY karyotype and mutations in the CBX2 gene. Am J Hum Genet. 2009;84(5):658-63.
- Umehara F, Tate G, Itoh K, Yamaguchi N, Douchi T, Mitsuya T, Osame M. A novel mutation of desert hedgehog in a patient with 46,XY partial gonadal dysgenesis accompanied by minifascicular neuropathy. Am J Hum Genet. 2000;67(5):1302-5.
- Quinonez SC, Park JM, Rabah R, Owens KM, Yashar BM, Glover TW, Keegan CE. 9p partial monosomy and disorders of sex development: review and postulation of a pathogenetic mechanism. Am J Med Genet A. 2013;161a(8):1882-96.
- da Silva TE, Gomes NL, Lerário AM, Keegan CE, Nishi MY, Carvalho FM, et al. Genetic Evidence of the Association of DEAH-Box Helicase 37 Defects With 46,XY Gonadal Dysgenesis Spectrum. J Clin Endocrinol Metab. 2019;104(12):5923-34.
- Pellegrini M, Pantano S, Lucchini F, Fumi M, Forabosco A. Emx2 developmental expression in the primordia of the reproductive and excretory systems. Anat Embryol (Berl). 1997;196(6):427-33.
- Lang-Muritano M, Sproll P, Wyss S, Kolly A, Hürlimann R, Konrad D, Biason-Lauber A. Early-Onset Complete Ovarian Failure and Lack of Puberty in a Woman With Mutated Estrogen Receptor β (ESR2). J Clin Endocrinol Metab. 2018;103(10):3748-56.
- Bagheri-Fam S, Ono M, Li L, Zhao L, Ryan J, Lai R, et al. FGFR2 mutation in 46,XY sex reversal with craniosynostosis. Hum Mol Genet. 2015;24(23):6699-710.
- Lourenço D, Brauner R, Rybczynska M, Nihoul-Fékété C, McElreavey K, Bashamboo A. Loss-of-function mutation in GATA4 causes anomalies of human testicular development. Proc Natl Acad Sci U S A. 2011;108(4):1597-602.
- Callier P, Calvel P, Matevossian A, Makrythanasis P, Bernard P, Kurosaka H, et al. Loss of function mutation in the palmitoyl-transferase HHAT leads to syndromic 46,XY disorder of sex development by impeding Hedgehog protein palmitoylation and signaling. PLoS Genet. 2014;10(5):e1004340.
- Kunitomo M, Khokhar A, Kresge C, Edobor-Osula F, Pletcher BA. 46,XY DSD and limb abnormalities in a female with a de novo LHX9 missense mutation. Am J Med Genet A. 2020;182(12):2887-90.
- Fukami M, Wada Y, Miyabayashi K, Nishino I, Hasegawa T, Nordenskjöld A, et al. CXorf6 is a causative gene for hypospadias. Nat Genet. 2006;38(12):1369-71.
- Pearlman A, Loke J, Le Caignec C, White S, Chin L, Friedman A, et al. Mutations in MAP3K1 cause 46,XY disorders of sex development and implicate a common signal transduction pathway in human testis determination. Am J Hum Genet. 2010;87(6):898-904.
- Bardoni B, Zanaria E, Guioli S, Floridia G, Worley KC, Tonini G, et al. A dosage sensitive locus at chromosome Xp21 is involved in male to female sex reversal. Nat Genet. 1994;7(4):497-501.
- Bashamboo A, Eozenou C, Jorgensen A, Bignon-Topalovic J, Siffroi JP, Hyon C, et al. Loss of Function of the Nuclear Receptor NR2F2, Encoding COUP-TF2, Causes Testis Development and Cardiac Defects in 46,XX Children. Am J Hum Genet. 2018;102(3):487-93.
- Achermann JC, Ito M, Ito M, Hindmarsh PC, Jameson JL. A mutation in the gene encoding steroidogenic factor-1 causes XY sex reversal and adrenal failure in humans. Nat Genet. 1999;22(2):125-6.
- Guran T, Yesil G, Turan S, Atay Z, Bozkurtlar E, Aghayev A, et al. PPP2R3C gene variants cause syndromic 46,XY gonadal dysgenesis and impaired spermatogenesis in humans. Eur J Endocrinol. 2019;180(5):291-309.
- Parma P, Radi O, Vidal V, Chaboissier MC, Dellambra E, Valentini S, et al. R-spondin1 is essential in sex determination, skin differentiation and malignancy. Nat Genet. 2006;38(11):1304-9.
- Ayers KL, Eggers S, Rollo BN, Smith KR, Davidson NM, Siddall NA, et al. Variants in SART3 cause a spliceosomopathy characterised by failure of testis development and neuronal defects. Nat Commun. 2023;14(1):3403.
- Sutton E, Hughes J, White S, Sekido R, Tan J, Arboleda V, et al. Identification of SOX3 as an XX male sex reversal gene in mice and humans. J Clin Invest. 2011;121(1):328-41.
- Portnoi MF, Dumargne MC, Rojo S, Witchel SF, Duncan AJ, Eozenou C, et al. Mutations involving the SRY-related gene SOX8 are associated with a spectrum of human reproductive anomalies. Hum Mol Genet. 2018;27(7):1228-40.
- Croft B, Ohnesorg T, Hewitt J, Bowles J, Quinn A, Tan J, et al. Human sex reversal is caused by duplication or deletion of core enhancers upstream of SOX9. Nat Commun. 2018;9(1):5319.
- Falah N, Posey JE, Thorson W, Benke P, Tekin M, Tarshish B, et al. 22q11.2q13 duplication including SOX10 causes sex-reversal and peripheral demyelinating neuropathy, central dysmyelinating leukodystrophy, Waardenburg syndrome, and Hirschsprung disease. Am J Med Genet A. 2017;173(4):1066-70.
- Ahmad A, Siddiqui MA, Goyal A, Wangnoo SK. Is 46XX karyotype always a female? BMJ Case Rep. 2012;2012.
- Jäger RJ, Anvret M, Hall K, Scherer G. A human XY female with a frame shift mutation in the candidate testis-determining gene SRY. Nature. 1990;348(6300):452-4.
- Puffenberger EG, Hu-Lince D, Parod JM, Craig DW, Dobrin SE, Conway AR, et al. Mapping of sudden infant death with dysgenesis of the testes syndrome (SIDDT) by a SNP genome scan and identification of TSPYL loss of function. Proc Natl Acad Sci U S A. 2004;101(32):11689-94.
- Eozenou C, Gonen N, Touzon MS, Jorgensen A, Yatsenko SA, Fusee L, et al. Testis formation in XX individuals resulting from novel pathogenic variants in Wilms' tumor 1 (WT1) gene. Proc Natl Acad Sci U S A. 2020;117(24):13680-8.
- Hammes A, Guo JK, Lutsch G, Leheste JR, Landrock D, Ziegler U, et al. Two splice variants of the Wilms' tumor 1 gene have distinct functions during sex determination and nephron formation. Cell. 2001;106(3):319-29.
- Mandel H, Shemer R, Borochowitz ZU, Okopnik M, Knopf C, Indelman M, et al. SERKAL syndrome: an autosomal-recessive disorder caused by a loss-of-function mutation in WNT4. Am J Hum Genet. 2008;82(1):39-47.
- Biason-Lauber A, Konrad D, Navratil F, Schoenle EJ. A WNT4 mutation associated with Müllerian-duct regression and virilization in a 46,XX woman. N Engl J Med. 2004;351(8):792-8.
- Bashamboo A, Brauner R, Bignon-Topalovic J, Lortat-Jacob S, Karageorgou V, Lourenco D, et al. Mutations in the FOG2/ZFPM2 gene are associated with anomalies of human testis determination. Hum Mol Genet. 2014;23(14):3657-65.
- Harris A, Siggers P, Corrochano S, Warr N, Sagar D, Grimes DT, et al. ZNRF3 functions in mammalian sex determination by inhibiting canonical WNT signaling. Proc Natl Acad Sci U S A. 2018;115(21):5474-9.
- Miller WL. MECHANISMS IN ENDOCRINOLOGY: Rare defects in adrenal steroidogenesis. Eur J Endocrinol. 2018;179(3):R125-r41.
- Flück CE, Meyer-Böni M, Pandey AV, Kempná P, Miller WL, Schoenle EJ, Biason-Lauber A. Why boys will be boys: two pathways of fetal testicular androgen biosynthesis are needed for male sexual differentiation. Am J Hum Genet. 2011;89(2):201-18.
- Flück CE, Pandey AV. Steroidogenesis of the testis -- new genes and pathways. Ann Endocrinol (Paris). 2014;75(2):40-7.
- Kok RC, Timmerman MA, Wolffenbuttel KP, Drop SL, de Jong FH. Isolated 17,20-lyase deficiency due to the cytochrome b5 mutation W27X. J Clin Endocrinol Metab. 2010;95(3):994-9.
- Khattab A, Yuen T, Yau M, Domenice S, Frade Costa EM, Diya K, et al. Pitfalls in hormonal diagnosis of 17-beta hydroxysteroid dehydrogenase III deficiency. J Pediatr Endocrinol Metab. 2015;28(5-6):623-8.
- Burkhard FZ, Parween S, Udhane SS, Flück CE, Pandey AV. P450 Oxidoreductase deficiency: Analysis of mutations and polymorphisms. J Steroid Biochem Mol Biol. 2017;165(Pt A):38-50.
- Miller WL, Huang N, Pandey AV, Flück CE, Agrawal V. P450 oxidoreductase deficiency: a new disorder of steroidogenesis. Ann N Y Acad Sci. 2005;1061:100-8.
- Miller WL, Huang N, Flück CE, Pandey AV. P450 oxidoreductase deficiency. Lancet. 2004;364(9446):1663.
- Walsh PC, Madden JD, Harrod MJ, Goldstein JL, MacDonald PC, Wilson JD. Familial incomplete male pseudohermaphroditism, type 2. Decreased dihydrotestosterone formation in pseudovaginal perineoscrotal hypospadias. N Engl J Med. 1974;291(18):944-9.
- Imperato-McGinley J, Zhu YS. Androgens and male physiology the syndrome of 5alpha-reductase-2 deficiency. Mol Cell Endocrinol. 2002;198(1-2):51-9.
- Kang HJ, Imperato-McGinley J, Zhu YS, Rosenwaks Z. The effect of 5α-reductase-2 deficiency on human fertility. Fertil Steril. 2014;101(2):310-6.
- Nordenskjöld A, Ivarsson SA. Molecular characterization of 5 alpha-reductase type 2 deficiency and fertility in a Swedish family. J Clin Endocrinol Metab. 1998;83(9):3236-8.
- Bertelloni S, Baldinotti F, Baroncelli GI, Caligo MA, Peroni D. Paternity in 5α-Reductase-2 Deficiency: Report of Two Brothers with Spontaneous or Assisted Fertility and Literature Review. Sex Dev. 2019;13(2):55-9.
- Mendonca BB, Batista RL, Domenice S, Costa EM, Arnhold IJ, Russell DW, Wilson JD. Steroid 5α-reductase 2 deficiency. J Steroid Biochem Mol Biol. 2016;163:206-11.
- Hornig NC, Holterhus PM. Molecular basis of androgen insensitivity syndromes. Mol Cell Endocrinol. 2021;523:111146.
- Batista RL, Costa EMF, Rodrigues AS, Gomes NL, Faria JA, Jr., Nishi MY, et al. Androgen insensitivity syndrome: a review. Arch Endocrinol Metab. 2018;62(2):227-35.
- Shukla GC, Plaga AR, Shankar E, Gupta S. Androgen receptor-related diseases: what do we know? Andrology. 2016;4(3):366-81.
- Zhou ZX, Wong CI, Sar M, Wilson EM. The androgen receptor: an overview. Recent Prog Horm Res. 1994;49:249-74.
- The androgen receptor gene mutation world wide web server[Available from: http://androgendb.mcgill.ca/.
- Gottlieb B TM. Androgen Insensitivity Syndrome. 1999 Mar 24 [Updated 2017 May 11]In: GeneReviews® [Internet]. Seattle (WA): University of Washington, Seattle; 1993-2023. Available from: https://www.ncbi.nlm.nih.gov/books/NBK1429/.
- Gottlieb B, Beitel LK, Nadarajah A, Paliouras M, Trifiro M. The androgen receptor gene mutations database: 2012 update. Hum Mutat. 2012;33(5):887-94.
- Ahmed SF, Bashamboo A, Lucas-Herald A, McElreavey K. Understanding the genetic aetiology in patients with XY DSD. Br Med Bull. 2013;106:67-89.
- Hornig NC, Ukat M, Schweikert HU, Hiort O, Werner R, Drop SL, et al. Identification of an AR Mutation-Negative Class of Androgen Insensitivity by Determining Endogenous AR Activity. J Clin Endocrinol Metab. 2016;101(11):4468-77.
- Hornig NC, Rodens P, Dörr H, Hubner NC, Kulle AE, Schweikert HU, et al. Epigenetic Repression of Androgen Receptor Transcription in Mutation-Negative Androgen Insensitivity Syndrome (AIS Type II). J Clin Endocrinol Metab. 2018;103(12):4617-27.
- Knerr J, Werner R, Schwan C, Wang H, Gebhardt P, Grötsch H, et al. Formin-mediated nuclear actin at androgen receptors promotes transcription. Nature. 2023;617(7961):616-22.
- Mongan NP, Tadokoro-Cuccaro R, Bunch T, Hughes IA. Androgen insensitivity syndrome. Best Pract Res Clin Endocrinol Metab. 2015;29(4):569-80.
- Hughes IA, Werner R, Bunch T, Hiort O. Androgen insensitivity syndrome. Semin Reprod Med. 2012;30(5):432-42.
- El-Maouche D, Arlt W, Merke DP. Congenital adrenal hyperplasia. Lancet. 2017;390(10108):2194-210.
- Morel Y, Roucher F, Plotton I, Simard J, Coll M. 3β-hydroxysteroid dehydrogenase deficiency.Genetic steroid disorders: Elsevier; 2014. p. 99-110.
- White PC. Genetic Steroid Disorders: Chapter 3D. Steroid 11β-Hydroxylase Deficiency and Related Disorders: Elsevier Inc. Chapters; 2013.
- Khattab A, Haider S, Kumar A, Dhawan S, Alam D, Romero R, et al. Clinical, genetic, and structural basis of congenital adrenal hyperplasia due to 11β-hydroxylase deficiency. Proc Natl Acad Sci U S A. 2017;114(10):E1933-e40.
- Khattab A, Marshall I. Management of congenital adrenal hyperplasia: beyond conventional glucocorticoid therapy. Curr Opin Pediatr. 2019;31(4):550-4.
- Yau M, Gujral J, New MI. Congenital Adrenal Hyperplasia: Diagnosis and Emergency Treatment. In: Feingold KR, Anawalt B, Blackman MR, Boyce A, Chrousos G, Corpas E, et al., editors. Endotext. South Dartmouth (MA): MDText.com, Inc. Copyright © 2000-2023, MDText.com, Inc.; 2000.
- Goto M, Piper Hanley K, Marcos J, Wood PJ, Wright S, Postle AD, et al. In humans, early cortisol biosynthesis provides a mechanism to safeguard female sexual development. J Clin Invest. 2006;116(4):953-60.
- Harada N. Genetic analysis of human placental aromatase deficiency. J Steroid Biochem Mol Biol. 1993;44(4-6):331-40.
- Wilkins L, Jones HW, Jr., Holman GH, Stempfel RS, Jr. Masculinization of the female fetus associated with administration of oral and intramuscular progestins during gestation: non-adrenal female pseudohermaphrodism. J Clin Endocrinol Metab. 1958;18(6):559-85.
- Troisi R, Hatch EE, Palmer JR, Titus L, Robboy SJ, Strohsnitter WC, et al. Prenatal diethylstilbestrol exposure and high-grade squamous cell neoplasia of the lower genital tract. Am J Obstet Gynecol. 2016;215(3):322.e1-8.
- Stillman RJ. In utero exposure to diethylstilbestrol: adverse effects on the reproductive tract and reproductive performance and male and female offspring. Am J Obstet Gynecol. 1982;142(7):905-21.
- Kalfa N, Philibert P, Baskin LS, Sultan C. Hypospadias: interactions between environment and genetics. Mol Cell Endocrinol. 2011;335(2):89-95.
- Jorgensen A, Svingen T, Miles H, Chetty T, Stukenborg JB, Mitchell RT. Environmental Impacts on Male Reproductive Development: Lessons from Experimental Models. Horm Res Paediatr. 2023;96(2):190-206.
- Hurtado-Gonzalez P, Mitchell RT. Analgesic use in pregnancy and male reproductive development. Curr Opin Endocrinol Diabetes Obes. 2017;24(3):225-32.
- Giordano J, Prior HM, Bamforth JS, Walter MA. Genetic study of SOX9 in a case of campomelic dysplasia. Am J Med Genet. 2001;98(2):176-81.
- Granata T, Freri E, Caccia C, Setola V, Taroni F, Battaglia G. Schizencephaly: clinical spectrum, epilepsy, and pathogenesis. J Child Neurol. 2005;20(4):313-8.
- Kremen J, Chan YM. Genetic evaluation of disorders of sex development: current practice and novel gene discovery. Curr Opin Endocrinol Diabetes Obes. 2019;26(1):54-9.
- Sofatzis JA, Alexacos L, Skouteli HN, Tiniakos G, Padiatellis C. Malformed female genitalia in newborns with the VATER association. Acta Paediatr Scand. 1983;72(6):923-4.
- Ahmed SF, Achermann JC, Arlt W, Balen A, Conway G, Edwards Z, et al. Society for Endocrinology UK guidance on the initial evaluation of an infant or an adolescent with a suspected disorder of sex development (Revised 2015). Clin Endocrinol (Oxf). 2016;84(5):771-88.
- Davies JH, Cheetham T. Recognition and assessment of atypical and ambiguous genitalia in the newborn. Arch Dis Child. 2017;102(10):968-74.
- Baidya A, Basu AK, Bhattacharjee R, Biswas D, Biswas K, Chakraborty PP, et al. Diagnostic approach in 46, XY DSD: an endocrine society of bengal (ESB) consensus statement. J Pediatr Endocrinol Metab. 2023;36(1):4-18.
- Bever YV, Brüggenwirth HT, Wolffenbuttel KP, Dessens AB, Groenenberg IAL, Knapen M, et al. Under-reported aspects of diagnosis and treatment addressed in the Dutch-Flemish guideline for comprehensive diagnostics in disorders/differences of sex development. J Med Genet. 2020;57(9):581-9.
- Prader A. [Genital findings in the female pseudo-hermaphroditism of the congenital adrenogenital syndrome; morphology, frequency, development and heredity of the different genital forms]. Helv Paediatr Acta. 1954;9(3):231-48.
- Quigley CA, De Bellis A, Marschke KB, el-Awady MK, Wilson EM, French FS. Androgen receptor defects: historical, clinical, and molecular perspectives. Endocr Rev. 1995;16(3):271-321.
- van der Straaten S, Springer A, Zecic A, Hebenstreit D, Tonnhofer U, Gawlik A, et al. The External Genitalia Score (EGS): A European Multicenter Validation Study. J Clin Endocrinol Metab. 2020;105(3).
- Cao W, Ding X, Dong Z, Tang H. Reference Values for and Correlation Analysis of the Anogenital Distance of Chinese Han Full-Term Singleton Neonates. Front Pediatr. 2022;10:905421.
- Vanderbrink BA, Rink RC, Cain MP, Kaefer M, Meldrum KK, Misseri R, Karmazyn B. Does preoperative genitography in congenital adrenal hyperplasia cases affect surgical approach to feminizing genitoplasty? J Urol. 2010;184(4 Suppl):1793-8.
- Eggers S, Sadedin S, van den Bergen JA, Robevska G, Ohnesorg T, Hewitt J, et al. Disorders of sex development: insights from targeted gene sequencing of a large international patient cohort. Genome Biol. 2016;17(1):243.
- Baxter RM, Arboleda VA, Lee H, Barseghyan H, Adam MP, Fechner PY, et al. Exome sequencing for the diagnosis of 46,XY disorders of sex development. J Clin Endocrinol Metab. 2015;100(2):E333-44.
- Bacila IA, Lawrence NR, Badrinath SG, Balagamage C, Krone NP. Biomarkers in congenital adrenal hyperplasia. Clin Endocrinol (Oxf). 2023.
- Ye L, Zhao Z, Ren H, Wang W, Zhou W, Zheng S, et al. A Multiclassifier System to Identify and Subtype Congenital Adrenal Hyperplasia Based on Circulating Steroid Hormones. J Clin Endocrinol Metab. 2022;107(8):e3304-e12.
- Kamrath C, Friedrich C, Hartmann MF, Wudy SA. Metabotypes of congenital adrenal hyperplasia in infants determined by gas chromatography-mass spectrometry in spot urine. J Steroid Biochem Mol Biol. 2023;231:106304.
- Jha S, Turcu AF, Sinaii N, Brookner B, Auchus RJ, Merke DP. 11-Oxygenated Androgens Useful in the Setting of Discrepant Conventional Biomarkers in 21-Hydroxylase Deficiency. J Endocr Soc. 2021;5(2):bvaa192.
- Bileck A, Fluck CE, Dhayat N, Groessl M. How high-resolution techniques enable reliable steroid identification and quantification. J Steroid Biochem Mol Biol. 2019;186:74-8.
- Dhayat NA, Frey AC, Frey BM, d'Uscio CH, Vogt B, Rousson V, et al. Estimation of reference curves for the urinary steroid metabolome in the first year of life in healthy children: Tracing the complexity of human postnatal steroidogenesis. J Steroid Biochem Mol Biol. 2015;154:226-36.
- Enver EO, Vatansever P, Guran O, Bilgin L, Boran P, Turan S, et al. Adrenal steroids reference ranges in infancy determined by LC-MS/MS. Pediatr Res. 2022;92(1):265-74.
- Guibourdenche J, Lucidarme N, Chevenne D, Rigal O, Nicolas M, Luton D, et al. Anti-Müllerian hormone levels in serum from human foetuses and children: pattern and clinical interest. Mol Cell Endocrinol. 2003;211(1-2):55-63.
- Schmidt H, Schwarz HP. Serum concentrations of LH and FSH in the healthy newborn. Eur J Endocrinol. 2000;143(2):213-5.
- Johannsen TH, Main KM, Ljubicic ML, Jensen TK, Andersen HR, Andersen MS, et al. Sex Differences in Reproductive Hormones During Mini-Puberty in Infants With Normal and Disordered Sex Development. J Clin Endocrinol Metab. 2018;103(8):3028-37.
- Bertelloni S, Russo G, Baroncelli GI. Human Chorionic Gonadotropin Test: Old Uncertainties, New Perspectives, and Value in 46,XY Disorders of Sex Development. Sex Dev. 2018;12(1-3):41-9.
- Gomes NL, Batista RL, Nishi MY, Lerário AM, Silva TE, de Moraes Narcizo A, et al. Contribution of Clinical and Genetic Approaches for Diagnosing 209 Index Cases With 46,XY Differences of Sex Development. J Clin Endocrinol Metab. 2022;107(5):e1797-e806.
- Guerrero-Fernández J, González-Peramato P, Rodríguez Estévez A, Alcázar Villar MJ, Audí Parera L, Azcona San Julián MC, et al. Consensus guide on prophylactic gonadectomy in different sex development. Endocrinol Diabetes Nutr (Engl Ed). 2022;69(8):629-45.
- Patel V, Casey RK, Gomez-Lobo V. Timing of Gonadectomy in Patients with Complete Androgen Insensitivity Syndrome-Current Recommendations and Future Directions. J Pediatr Adolesc Gynecol. 2016;29(4):320-5.
- Sircili MH, e Silva FA, Costa EM, Brito VN, Arnhold IJ, Dénes FT, et al. Long-term surgical outcome of masculinizing genitoplasty in large cohort of patients with disorders of sex development. J Urol. 2010;184(3):1122-7.
- Yan M, Dilihuma J, Luo Y, Reyilanmu B, Shen Y, Mireguli M. Novel Compound Heterozygous Variants in the LHCGR Gene in a Genetically Male Patient with Female External Genitalia. J Clin Res Pediatr Endocrinol. 2019;11(2):211-7.
- Berg JS, French SL, McCullough LB, Kleppe S, Sutton VR, Gunn SK, Karaviti LP. Ethical and legal implications of genetic testing in androgen insensitivity syndrome. J Pediatr. 2007;150(4):434-8.
- Wisniewski AB. Complete Androgen Insensitivity Syndrome: Long-Term Medical, Surgical, and Psychosexual Outcome. Journal of Clinical Endocrinology & Metabolism. 2000;85(8):2664-9.
- Bianco S, Agrifoglio V, Mannino F, Cefalù E, Cittadini E. Successful pregnancy in a pure gonadal dysgenesis with karyotype 46,XY patient (Swyer's syndrome) following oocyte donation and hormonal treatment. Acta Eur Fertil. 1992;23(1):37-8.
- Hines M, Ahmed SF, Hughes IA. Archives of Sexual Behavior. 2003;32(2):93-101.
- Park S, Ha SH, Kim KS. Long-term follow-up after feminizing genital reconstruction in patients with ambiguous genitalia and high vaginal confluence. Journal of Korean medical science. 2011;26(3):399-403.
- Sircili MHP, de Queiroz e Silva FA, Costa EMF, Brito VN, Arnhold IJP, Dénes FT, et al. Long-Term Surgical Outcome of Masculinizing Genitoplasty in Large Cohort of Patients With Disorders of Sex Development. Journal of Urology. 2010;184(3):1122-7.
- Wisniewski AB, Migeon CJ, Gearhart JP, Rock JA, Berkovitz GD, Plotnick LP, et al. Congenital Micropenis: Long-Term Medical, Surgical and Psychosexual Follow-Up of Individuals Raised Male or Female. Hormone Research in Paediatrics. 2001;56(1-2):3-11.
- Mendonca BB, Costa EM, Belgorosky A, Rivarola MA, Domenice S. 46,XY DSD due to impaired androgen production. Best Pract Res Clin Endocrinol Metab. 2010;24(2):243-62.
- Batista RL, Mendonca BB. Integrative and Analytical Review of the 5-Alpha-Reductase Type 2 Deficiency Worldwide. Appl Clin Genet. 2020;13:83-96.
- Bonnet E, Winter M, Mallet D, Plotton I, Bouvattier C, Cartigny M, et al. Changes in the clinical management of 5α-reductase type 2 and 17β-hydroxysteroid dehydrogenase type 3 deficiencies in France. Endocr Connect. 2023;12(3).
- Duranteau L, Rapp M, van de Grift TC, Hirschberg AL, Nordenskjöld A. Participant- and Clinician-Reported Long-Term Outcomes After Surgery in Individuals with Complete Androgen Insensitivity Syndrome. J Pediatr Adolesc Gynecol. 2021;34(2):168-75.
- Dessens AB, Slijper FME, Drop SLS. Gender Dysphoria and Gender Change in Chromosomal Females with Congenital Adrenal Hyperplasia. Archives of Sexual Behavior. 2005;34(4):389-97.
- Long DN, Wisniewski AB, Migeon CJ. Gender Role Across Development in Adult Women with Congenital Adrenal Hyperplasia Due to 21-Hydroxylase Deficiency. Journal of Pediatric Endocrinology and Metabolism. 2004;17(10).
- Wisniewski AB, Migeon CJ, Malouf MA, Gearhart JP. PSYCHOSEXUAL OUTCOME IN WOMEN AFFECTED BY CONGENITAL ADRENAL HYPERPLASIA DUE TO 21-HYDROXYLASE DEFICIENCY. Journal of Urology. 2004;171(6 Part 1):2497-501.
- Lee PA, Houk CP. Outcome Studies Among Men with Micropenis. Journal of Pediatric Endocrinology and Metabolism. 2004;17(8).
- Lee PA, Houk CP, Husmann DA. Should Male Gender Assignment be Considered in the Markedly Virilized Patient With 46,XX and Congenital Adrenal Hyperplasia? Journal of Urology. 2010;184(4S):1786-92.
- de Jesus LE, Costa EC, Dekermacher S. Gender dysphoria and XX congenital adrenal hyperplasia: how frequent is it? Is male-sex rearing a good idea? Journal of Pediatric Surgery. 2019;54(11):2421-7.
- Crissman HP, Warner L, Gardner M, Carr M, Schast A, Quittner AL, et al. Children with disorders of sex development: A qualitative study of early parental experience. International journal of pediatric endocrinology. 2011;2011(1):10-.
- Yau M, Khattab A, New MI. Prenatal Diagnosis of Congenital Adrenal Hyperplasia. Endocrinology and Metabolism Clinics of North America. 2016;45(2):267-81.
- Van't Westeinde A, Karlsson L, Messina V, Wallensteen L, Brösamle M, Dal Maso G, et al. An update on the long-term outcomes of prenatal dexamethasone treatment in congenital adrenal hyperplasia. Endocr Connect. 2023;12(4).
- Kazmi D, Bailey J, Yau M, Abu-Amer W, Kumar A, Low M, Yuen T. New developments in prenatal diagnosis of congenital adrenal hyperplasia. The Journal of Steroid Biochemistry and Molecular Biology. 2017;165:121-3.
- Maher JY, Gomez-Lobo V, Merke DP. The management of congenital adrenal hyperplasia during preconception, pregnancy, and postpartum. Rev Endocr Metab Disord. 2023;24(1):71-83.
- Fedele D, Kirk K, Wolfe-Christensen C, Phillips T, Mazur T, Mullins L, et al. Primary Caregivers of Children Affected by Disorders of Sex Development: Mental Health and Caregiver Characteristics in the Context of Genital Ambiguity and Genitoplasty. International Journal of Pediatric Endocrinology. 2010;2010(1):690674.
- Hullmann SE, Fedele DA, Wolfe-Christensen C, Mullins LL, Wisniewski AB. Differences in adjustment by child developmental stage among caregivers of children with disorders of sex development. International journal of pediatric endocrinology. 2011;2011(1):16-.
- Kirk KD, Fedele DA, Wolfe-Christensen C, Phillips TM, Mazur T, Mullins LL, et al. Parenting Characteristics of Female Caregivers of Children Affected by Chronic Endocrine Conditions: A Comparison Between Disorders of Sex Development and Type 1 Diabetes Mellitus. Journal of Pediatric Nursing. 2011;26(6):e29-e36.
- Wolfe-Christensen C, Fedele DA, Kirk K, Phillips TM, Mazur T, Mullins LL, et al. Degree of External Genital Malformation at Birth in Children with a Disorder of Sex Development and Subsequent Caregiver Distress. Journal of Urology. 2012;188(4S):1596-600.
- Wisniewski AB, Batista RL, Costa EMF, Finlayson C, Sircili MHP, Dénes FT, et al. Management of 46,XY Differences/Disorders of Sex Development (DSD) Throughout Life. Endocrine Reviews. 2019;40(6):1547-72.
- Magritte E. Working together in placing the long term interests of the child at the heart of the DSD evaluation. Journal of Pediatric Urology. 2012;8(6):571-5.