ABSTRACT
The 46,XY differences of sex development (46,XY DSD) can result either from decreased synthesis of testosterone and/or DHT or from impairment of androgen action. 46,XY DSD are characterized by micropenis, atypical or female external genitalia, caused by incomplete intrauterine masculinization with or without the presence of Müllerian structures. Male gonads are identified in the majority of 46,XY DSD patients, but in some of them no gonadal tissue is found. Complete absence of virilization results in normal female external genitalia and these patients generally seek medical attention at pubertal age, due to the absence of breast development and/or primary amenorrhea. A careful clinical evaluation of the neonate is essential because most DSD patients could be recognized in this period and prompt diagnosis allows a better therapeutic approach. Family and prenatal history, complete physical examination and assessment of genital anatomy are the first steps for a correct diagnosis. The diagnostic evaluation of DSD includes hormone measurements (assessment of Leydig and Sertoli cell function), imaging (ultrasonography is always the first and often the most valuable imaging modality in DSD patients’ investigation), cytogenetic, and molecular studies. Endoscopic and laparoscopic exploitation and/or gonadal biopsy are required in very few cases. Psychological evaluation is of crucial importance to treat DSD patients. Every couple that has a child with atypical genitalia must be assessed and receive counseling by an experienced psychologist, specialized in gender identity, who must act as soon as the diagnosis is suspected, and then follow the family periodically, more frequently during the periods before and after genitoplasty. Parents must be well informed by the physician and psychologist about normal sexual development. A simple, detailed, and comprehensive explanation about what to expect regarding integration in social life, sexual activity, need of hormonal and surgical treatment and the likely possibility or not of fertility according to the sex of rearing, should also be discussed with the parents before the assignment of final social sex. Optimal care of DSD patients begins in the newborn period and sometimes in prenatal life and requires a multidisciplinary team. Most of the well-treated DSD patients present a normal quality of life in adulthood.
INTRODUCTION
Male phenotypic development is a 2-step process: 1) testis formation from the primitive gonad (sexual determination) and 2) internal and external genitalia differentiation by action of factors secreted by the fetal testis (sexual differentiation). The first step is very complex and involves interplay of several transcription factors and signaling cells (1-3). Dosage imbalances in genes involved in DSD (deletions or duplication) have also been identified as a cause of these developmental differences (Fig. 1).
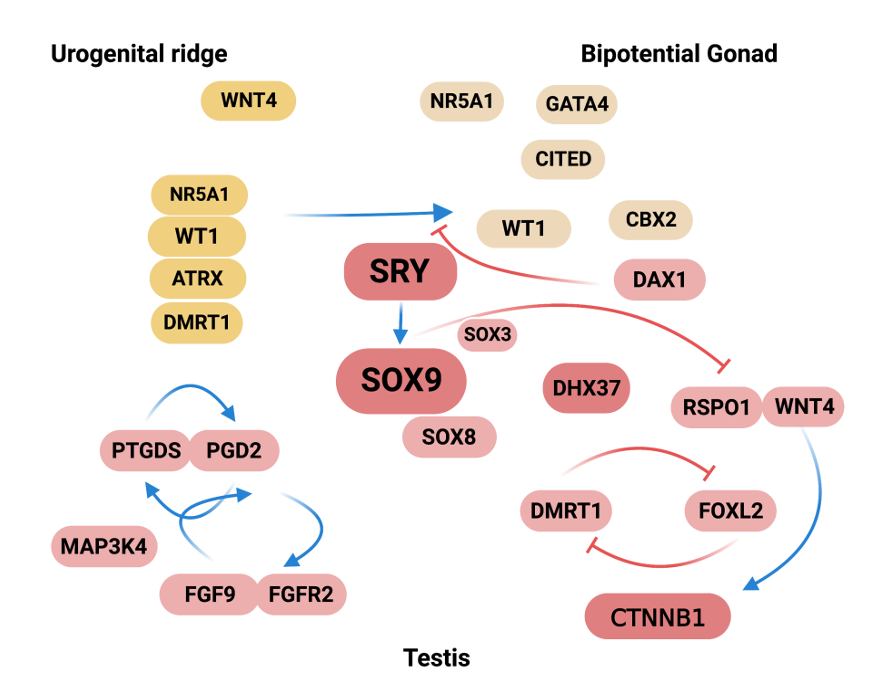
Figure 1. Summary of the molecular events in sex determination indicating the genes in which molecular defects can cause gonadal disorders in animal models. Some of these disorders were confirmed in humans. Nr5a1, Wnt4 and Wt1 are expressed in the urogenital ridge whose development results in formation of the gonads, kidneys, and adrenal cortex. Several genes, Wt1, Nr5a1, M33 (CBX2 mouse homologue), Lhx9, Lim1, Gata4/Fog2, Dmrt1, Emx2 and Cited are expressed in the bipotential gonad. Nr5a1 up-regulates Cbx2 expression that is required for upregulation of the Sry gene. Nr5a1 and Wt1 up-regulate Sry expression in pre-Sertoli cells and Sry initiates male gonad development. Sry strongly up-regulates Sox9 in Sertoli cells. Sox9 up-regulates Fgf9 and Fgf9 maintains Sox9 expression, forming a positive feed-forward loop in XY gonads. The balance between Fgf9 and Rspo1/Wnt4 signals is shifted in favor of Fgf9, establishing the male pathway. If Wnt4/Rspo1 is overexpressed activating the β-catenin pathway, this system blocks Fgf9 and disrupts the feed-forward loop between Sox9 and Fgf9. Pdg2 signaling up-regulates Sox9 and Sox9 activate Ptgds. Sox9 establishes a feed-forward loop with the Pgd2. Sox9 inhibits beta-catenin-mediated Wnt signaling. Overexpression in either Dax1 (locus DSS) or Rspo1/Wnt4 antagonizes testis formation. On the other hand, Dax1 regulates the development of peritubular myoid cells and the formation of testicular cords. Dmrt1 has recently been shown to be required for the maintenance of gonadal sex and to prevent female reprogramming in postnatal testis, Cbx2 directly or indirectly repress ovarian development. Dhx37 has critical roles in early human testis determination and also in the maintenance of testicular tissue.
The second step, male sex differentiation, is a more straightforward process. Mesonephric (Wolffian) and paramesonephric (Mullerian) ducts are present in both, male and female fetuses, and originate from the anterolateral epithelium of the urogenital ridge. Anti Müllerian hormone (AMH) secreted by the testicular Sertoli cells acts on its receptor in the Müllerian ducts to cause their regression. Testosterone secreted by the testicular Leydig cells acts on the androgen receptor in the Wolffian ducts to induce the formation of epididymis, deferent ducts and seminal vesicles (Fig. 2) (4). The external genitalia of the fetus derive from mesenchyme cells from the primitive streak. Under androgen stimuli male fetal urethral folds, genital tubercle and genital swellings give rise to corpus spongiosum and primitive urethra, phallus, and scrotal swellings respectively. This process is mediated by testosterone and its further reduced dihydrotestosterone (DHT), which acts on the androgen receptor of the prostate and external genitalia leading to their masculinization (5,6) (Figs. 3 - 8).
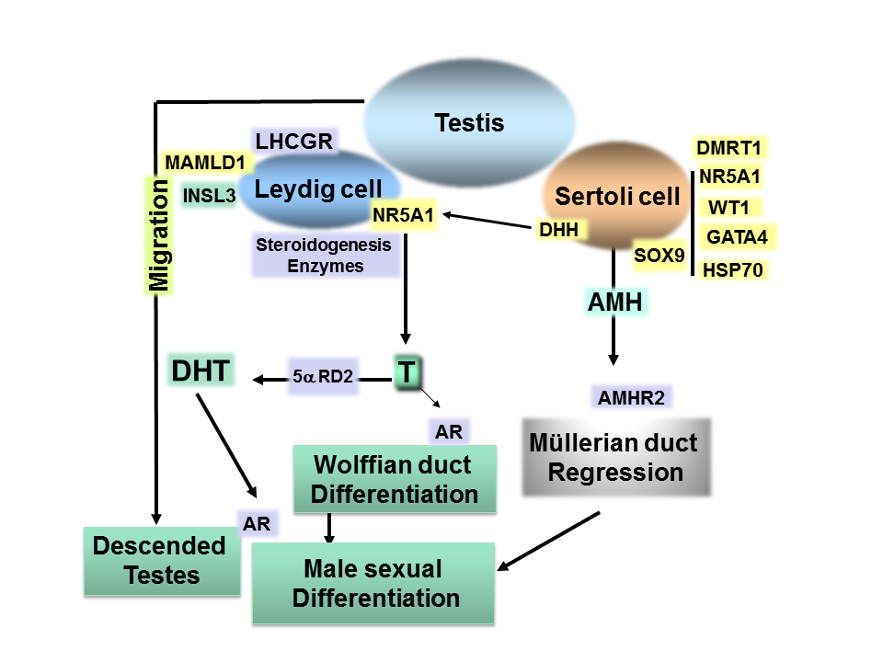
Figure 2. Summary of the molecular events in sex differentiation indicating the genes in which molecular defects cause 46,XY DSD in humans. After testis determination, hormones produced by the male gonad induce the differentiation of internal and external genitalia acting on their specific receptor. The regulation of AMH gene requires cooperative interaction between SOX9 and NR5A1, WT1, GATA4 and HSP70 at the AMH promoter. Combined expression of DHH, MAMLD1 and NR5A1 is required for Leydig cell development. NR5A1 regulates gonadal steroidogenesis. The Leydig cells also produce INSL3, which causes the testes to descend to the scrotum.
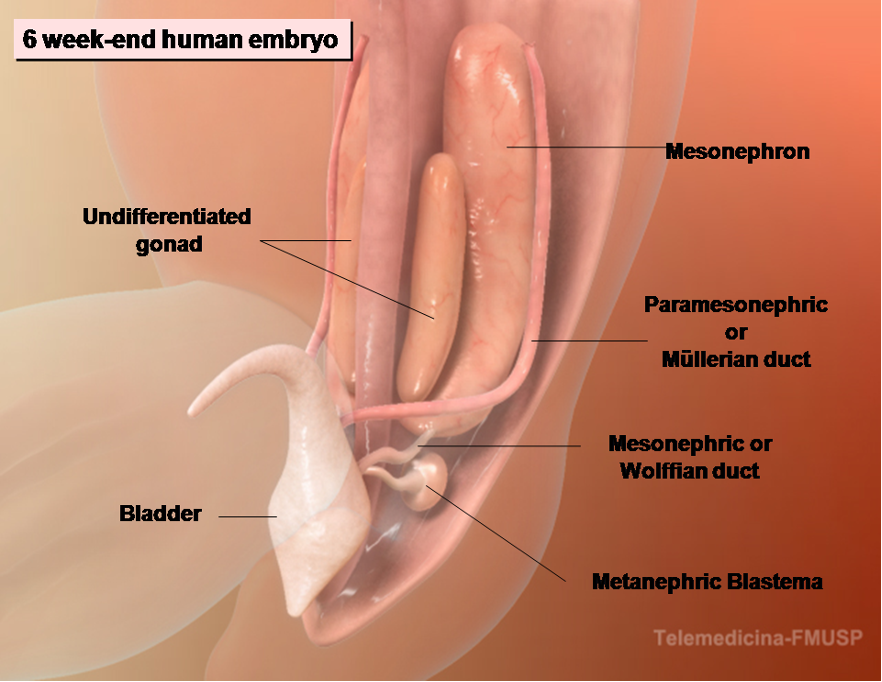
Figure 3. The development of male internal genitalia in the human embryo. The 6-wk-end embryo is equipped with both male and female genital ducts derived from the mesonephrons.
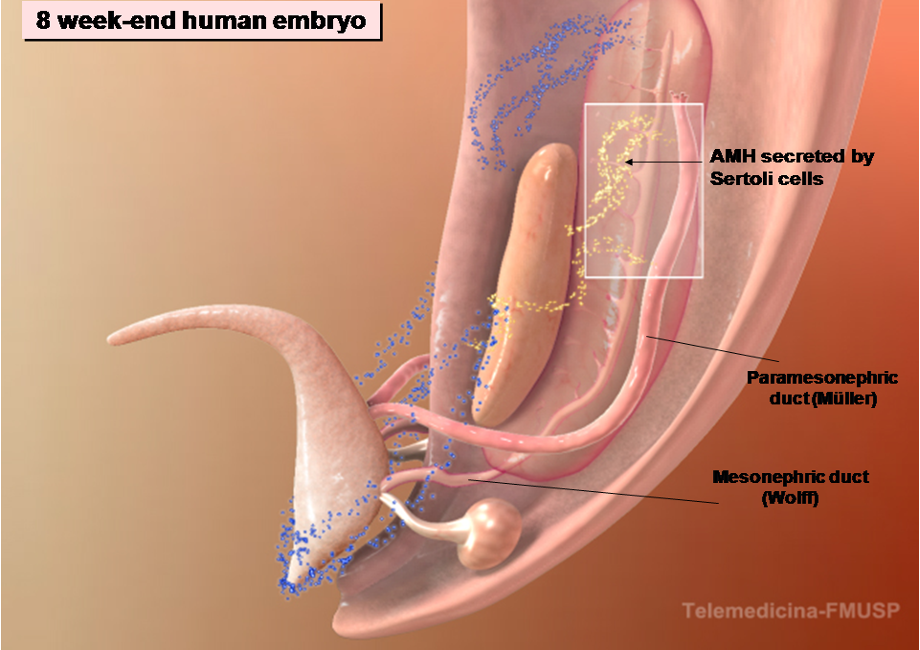
Figure 4. The development of male internal genitalia in the human embryo. The regression of the Müllerian ducts is mediated by the action of AMH secreted by the fetal Sertoli cells.
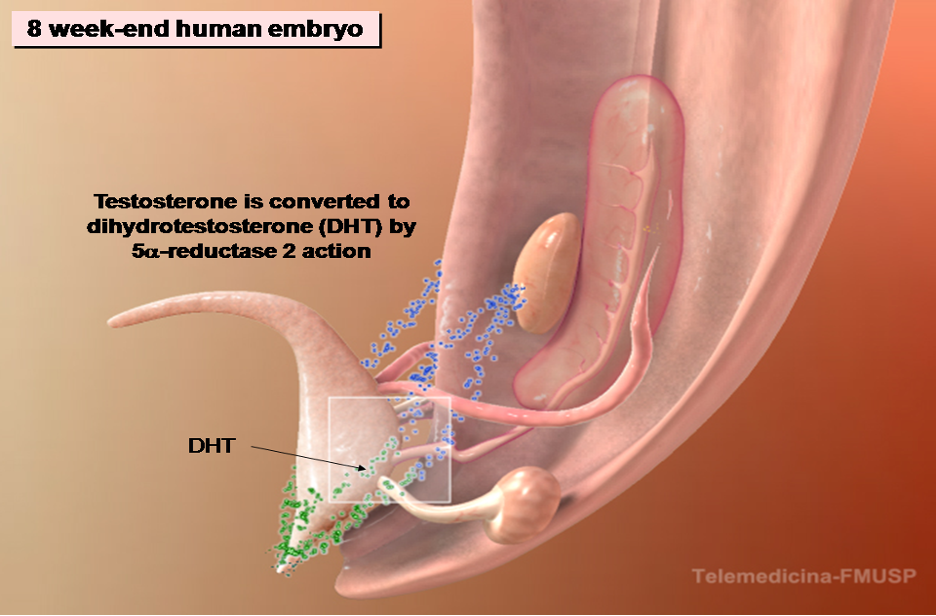
Figure 5. The development of male internal genitalia in the human embryo. The stabilization and differentiation of the Wolffian ducts are mediated by testosterone synthesized by the fetal Leydig cells. The enzyme 5α-reductase 2 converts testosterone to dihydrotestosterone (DHT). The Wolffian ducts differentiate into epididymis, vas deferens and seminal vesicles. DHT contributes to prostate differentiation.
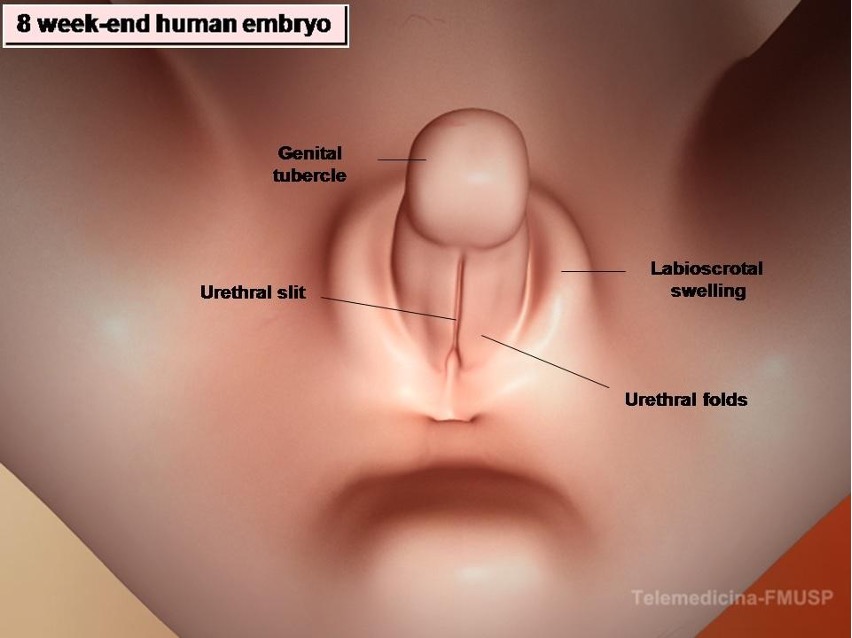
Figure 6. Development of male external genitalia in the human embryo. At the 8-wk-end embryo the external genitalia of both sexes are identical and have the capacity to differentiate in both directions: male or female. DHT stimulates growth of the genital tubercle and induces fusion of urethral folds and labioscrotal swellings. It also inhibits growth of the vesicovaginal septum, preventing development of the vagina.
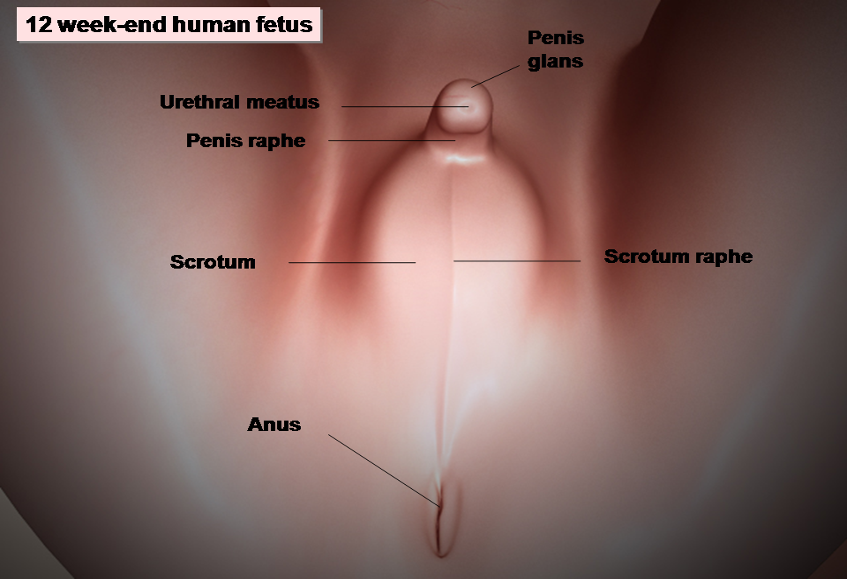
Figure 7. Development of male external genitalia in the human embryo. At the 12-week-end embryo the male external genitalia are entirely formed.
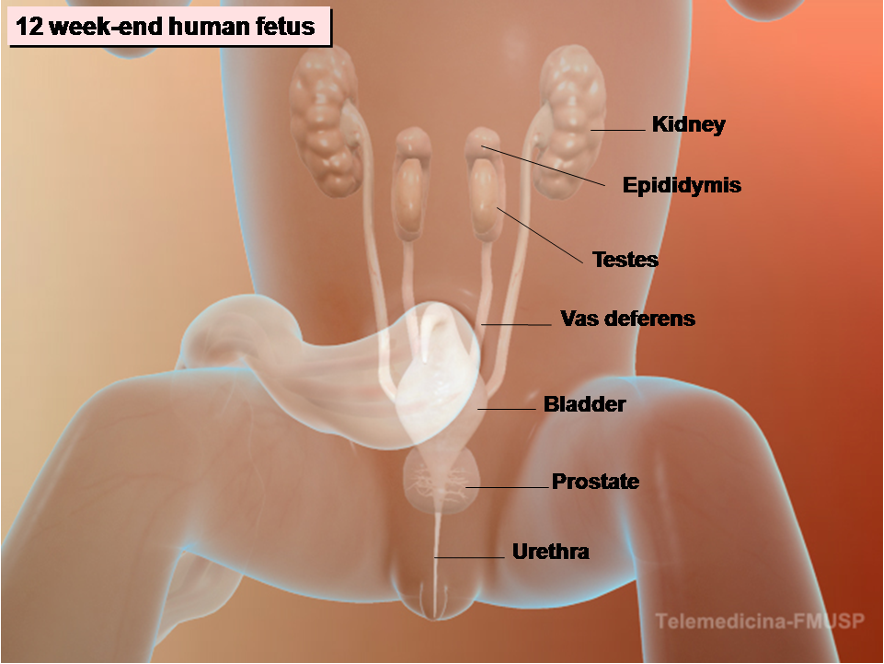
Figure 8. Development of male internal and external genitalia in the human embryo. At the 12-week-end embryo both internal and external genitalia are completely formed.
The term differences of sex development (DSD) include- congenital conditions in which development of chromosomal, or gonadal or anatomical sex is atypical. This nomenclature has been proposed to replace terms such as intersex, pseudo-hermaphroditism and sex reversal (6,7). These terms, previously used to describe the differences of sex development, are potentially offensive to the patients and the consensus on the management of intersex disorders recommends a new nomenclature that will be followed in this chapter (6). The proposed changes in terminology aim to integrate upcoming advances in molecular genetics in the most recent DSD classification (8).
The 46,XY DSDs are characterized by micro-penis, atypical or female external genitalia, caused by incomplete intrauterine masculinization with or without the presence of Müllerian structures. Male gonads are identified in the majority of 46,XY DSD patients, but in some of them no gonadal tissue is found. Complete absence of virilization results in normal female external genitalia and these patients generally seek medical attention at pubertal age, due to the absence of breast development and/or primary amenorrhea. 46,XY DSD can result either from decreased synthesis of testosterone or DHT or from impairment of androgen action (9,10). Our proposal classification of 46,XY DSD is displayed in Table 1.
Table 1. Classification of 46,XY DSD
|
46,XY DSD DUE TO ABNORMALITIES OF GONADAL DEVELOPMENT
Gonadal agenesis
Gonadal dysgenesis - complete and partial forms
|
46,XY DSD ASSOCIATED WITH CHOLESTEROL SYNTHESIS DEFECTS
Smith-Lemli-Opitz syndrome
|
46,XY DSD DUE TO TESTOSTERONE PRODUCTION DEFECTS
|
Impaired Leydig cell differentiation (LHCGR defects)
|
Complete and partial forms
|
Enzymatic defects in testosterone synthesis
Defects in adrenal and testicular steroidogenesis
STAR deficiency
|
P450scc deficiency
|
3-β-hydroxysteroid dehydrogenase II deficiency
|
17α-hydroxylase and 17,20 lyase deficiency
|
P450 oxidoreductase defect (electron transfer disruption)
|
Defects in testicular steroidogenesis
|
Isolated 17,20-lyase deficiency
Cytochrome b5 defect (allosteric factor for P450c17 and POR interaction)
17β-hydroxysteroid dehydrogenase III deficiency
|
Alternative pathway to DHT
|
3α- hydroxysteroid dehydrogenase deficiency due to AKR1C2 and AKR1C4 defects
|
46,XY DSD DUE TO DEFECTS IN TESTOSTERONE METABOLISM
5α-reductase type 2 deficiency
|
46,XY DSD DUE TO DEFECTS IN ANDROGEN ACTION
|
Androgen insensitivity syndrome
Complete and partial forms
|
46,XY DSD DUE TO PERSISTENCE OF MÜLLERIAN DUCTS
Defect in AMH synthesis
|
Defect in AMH receptor
|
CONGENITAL NON-GENETIC 46,XY DSD
Maternal intake of endocrine disruptors
Associated with impaired prenatal growth
|
46,XY OVOTESTICULAR DSD
|
NON-CLASSIFIED FORMS
Hypospadias
46,XY gender dysphoria
|
INVESTIGATION OF DSD PATIENTS
Optimal care of patients with DSD requires a multidisciplinary team and begins in the newborn period. A careful clinical evaluation of the neonate is essential because most DSD patients could be recognized in this period and prompt diagnosis allows a better therapeutic approach. Family and prenatal history, complete physical examination and assessment of genital anatomy are the first steps for a correct diagnosis. The diagnostic evaluation of DSD includes hormone measurements, imaging, cytogenetic, and molecular studies (11). In very few cases, endoscopic and laparoscopic exploitation and/or gonadal biopsy are required (12).
The endocrinological evaluation of 46,XY DSD infants includes assessment of testicular function by basal measurements of LH, FSH, inhibin B, anti-Mullerian hormone (AMH), and steroids. AMH and inhibin B are useful markers of the presence of Sertoli cells and their assessment could help in the diagnosis of testis determination disorders. In boys with bilateral cryptorchidism serum AMH and inhibin B correlate with the presence of testicular tissue and undetectable values are highly suggestive of absence of testicular tissue (13,14).
In minipuberty and in postpubertal patients with testosterone synthesis defects, the diagnosis is made through basal steroid levels. Testosterone levels are low and steroids upstream from the enzymatic blockage are elevated. This pattern can be confirmed by an hCG stimulation test, which increases the accumulation of steroids before the enzymatic blockage, with a slight elevation of testosterone. In prepubertal individuals, an hCG stimulation test is essential for the diagnosis, since basal levels are not altered.
There are several hCG stimulation protocols and normative data must be established for each of them. We established a normal testosterone response 72 and 96 hours after the last of 4 doses of hCG, 50-100 U/kg body weight, given via intramuscular every 4 days in boys with cryptorchidism but otherwise normal external genitalia: testosterone peak levels reached 391 ± 129 ng/dL and we consider a subnormal response a value <130 ng/dL (equivalent to -2 SD) (15).
Imaging evaluation is indicated in the neonatal period when atypical genitalia are identified. If apparent female genitalia with clitoral hypertrophy, posterior labial fusion, foreshortened vulva with single opening or inguinal/labial mass is present, imaging studies may also be performed. A family history of DSD and later presentations as abnormal puberty or primary amenorrhea, cyclic hematuria in a male, and inguinal hernia in a female also require an imaging evaluation.
Ultrasonography is always the first and often the most valuable imaging modality in DSD patients’ investigation. Ultrasound shows the presence or absence of Müllerian structures at all ages and can locate the gonads and characterize their echo texture. This exam can also identify associated malformations such as kidney abnormalities (16).
Genitography and cystourethrography can display the type of urethra, the presence of vagina, cervix, and urogenital sinus. MRI contributes to accurate morphologic evaluation of Mullerian duct structures, the gonads, and the development of the phallus, all of which are essential for appropriate gender assignment and planning of surgical reconstruction (17).
CYTOGENETIC AND MOLECULAR INVESTIGATION
The routine use of genetic testing for reaching a diagnosis in XY DSD is increasingly playing an important role in the diagnostic process. A wide range of techniques may be used, each one having a different investigative application and genetic resolution (18,19).
More than 75 genes involved in gonadal development and/or sex hormone biosynthesis/action are known causes of DSDs and the molecular methodologies have contributed to identify already known as well as novel causes of DSD. These results have led to the adoption of molecular tests into clinical practice for diagnosis and genetic counseling, reducing the need of hormonal and imaging tests to reach the correct diagnosis (20). Advances in molecular biological techniques for diagnosing DSD are reviewed in recent publications (18,19).
Chromosomal Analysis
Early identification of chromosomal regions and candidate-genes involved in the DSD etiology
were established by finding microscopically visible structural changes in the karyotype, using conventional cytogenetic techniques. Many of them were achieved by positional cloning and linkage analysis, which are not widely used tools.
Although conventional karyotyping is still used frequently in routine clinical diagnosis, faster molecular cytogenetic techniques that do not require cell culture can be employed. Array techniques [array comparative genomic hybridization (aCGH) and single-nucleotide polymorphism (SNP) array] are all capable to identify submicroscopic genome imbalance / copy number variation (CNV), as small as 10 KB (CNVs between 10 kb and 5 Mb in size), and which may affect several genes, in patients with an apparently normal karyotype (21,22).
CNV affecting coding sequences or regulatory elements of critical dosage-sensitive genes are known causes of DSDs (23-26). Novel DSD candidate chromosomic regions and genes, with potential roles in sex determination and DSD, such as SUPT3H, C2ORF80, KANK1, ADCY2, VAMP7 and ZEB2, have been also identified by array studies, many of them waiting for further validation (25,27).
Array techniques can diagnose pathogenic CNV in almost 30% of syndromic DSD patients as a single method (27,28). Thus, a CGH or SNP-array was proposed as the first genomic test to investigate this group of DSD patients.
Sequencing Analysis
Among the genetic tests, many use a candidate-gene approach (Sanger sequencing), while targeted DSD gene panels, wider whole-exome (WES) and whole-genome (WGS) scale are high-throughput screening technologies, in which multiple short DNA target sequences are analyzed to identified the presence of allelic variants (29).
Sanger sequencing is often the method of choice if a specific genetic condition is highly suspected by an established clinical and hormonal diagnosis. AR and SRD5A2, in addition to almost all testosterone synthesis defects, are the most requested genes in 46,XY DSD to be sequenced using this approach (20).
The superiority of targeted DSD gene panel tests, that can evaluate simultaneously several and non-standard sets of genes, over single-gene testing approaches is well established, especially considering time and cost-effectiveness (26,30).
Whole-Exome Sequencing (WES) and Whole-Genome Sequencing (WGS) are also based on short-read sequencing. They present a clear improvement over single-gene testing in providing clinical diagnosis for DSD. The advantage of WES/WGS is the potential to identify new DSD-related genes in the research setting. On the other hand, WGS has more consistent coverage of gene sequences throughout the genome, including the non-coding regions and so it has the potential to provide a much higher diagnostic yield than WES (25,31).
Nevertheless, WES and WGS require significant bioinformatic resources and are expensive strategies; consequently, their application for first-line diagnostic investigation in many clinical settings are still limited (18,32).
The target DNA can also be read in longer fragments (several kb). The main advantage of using long-reads during the process is that repetitive elements and complex structural variants can often be resolved to a greater extent than in assemblies generated from short-read sequencing (18). Long-read sequencing offers a potential solution to genome-wide short tandem repeats analysis, which are highly variable elements, which play a pivotal role in the regulation of gene expression.
Studies in animal models have suggested the involvement of epigenetic regulation in the process of gonadal formation, reinforcing a probable role of epigenetic variation in the etiology of DSD (33).
Careful selection of the genetic test indicated for each condition remains important for a good clinical practice (Figure 9).
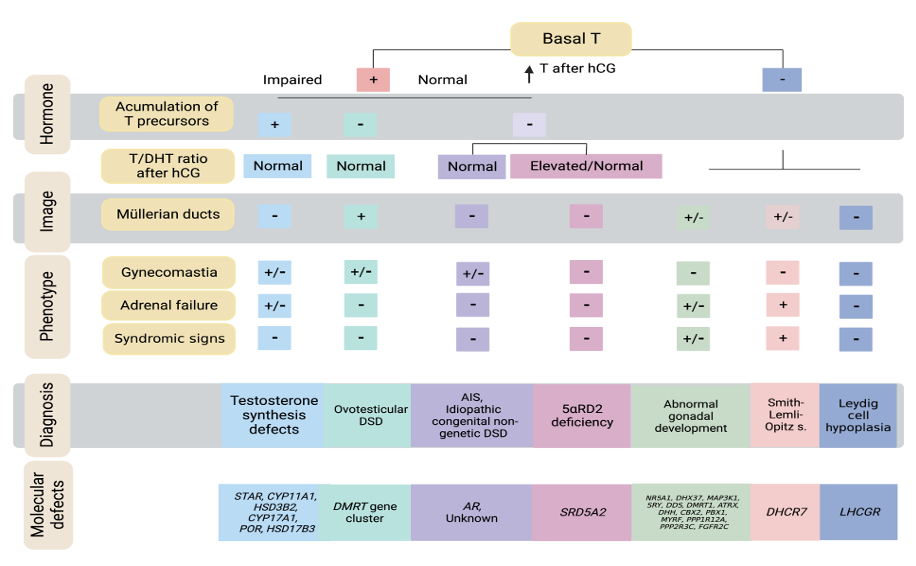
Figure 9. Algorithm for 46,XY DSD diagnosis
46,XY DSD DUE TO ABNORMALITIES IN GONADAL DEVELOPMENT
Uncountable allelic variants identified in several genes involved in the process of human gonadal determination have been associated with 46,XY gonadal dysgenesis. They will be described according to the period of gene expression in gonadal determination.
Gonadal Determination and Differentiation
The intermediate mesoderm is the primary embryonic tissue at gastrulation that gives rise to the urogenital ridge. This, in turns, is going to derive the primitive gonad from a condensation of the medioventral region of the urogenital ridge. The primitive gonad separates from the adrenal primordium at about 5 weeks but remains bipotential until the 6thweek after conception. Mammals sex determination is a complex process, which involves many genes acting in networks. Several genes have been involved in the development of the urogenital ridge, including Emx2, Lim1, Lhx9, Wt1, Gata-4/Fog2, Nr5a1/Sf1. Although knockout models of these genes produce abnormal gonads in mice, not all of them have been implicated in the human gonadal dysgenesis etiology.
To date, Emx2 null mice have absent kidneys, ureters, gonads and genital tracts and have developmental abnormalities of the brain (34). In humans, variants in EMX2 have been found in patients with schizencephaly (a rare condition in which a person is born with clefts in the brain that are filled with liquor) but no gonadal phenotypes have been described. WT1, NR5A1 and NR0B1/DAX1 are well known genes that are critical for the formation of the urogenital ridge in humans. The products of WT1 are essential for both gonadal and renal formation (35) whereas NR5A1/SF1 protein is essential for gonadal and adrenal formation (36,37). NR0B1/DAX1 is also essential for gonadal and adrenal differentiation and when mutated, results in congenital adrenal hypoplasia and hypogonadotropic hypogonadism (38).
After the formation of the bipotential gonad, by the 6th week after conception, in 46, XY individuals, the expression of the testis-determining gene Sry, which is transcriptionally regulated by the expression of Wt1 (39) and GATA Binding Protein 4 (Gata4), its cofactor the Friend-of-GATA (Fog2) (40) and chromobox protein homolog 2 (Cbx2) (41) trigger the gonadal masculinizing fate process. In the mammalian male embryo, the first molecular signal of sex determination is the expression of Sry within a subpopulation of somatic cells of the indifferent genital ridge (42). The transient expression of Sry drives the initial differentiation of pre-Sertoli cells that would otherwise follow a female pathway, becoming granulosa cells. Once Sry expression begins, it initiates the cascade of gene interactions and cellular events that direct the formation of a testis from the undifferentiated fetal gonad. So, pre-Sertoli cells proliferate, polarize and aggregate around the germ cells to define the testes cords. Migration of cells into the gonad from the mesonephros or the coelomic epithelium is subsequently induced by signals emanating from the pre-Sertoli cells. Peritubular myoid cells surround the testes cords and cooperate with pre-Sertoli cells to deposit the basal lamina and further define the testis cords. Signaling molecules produced by the pre-Sertoli cells promote the differentiation of somatic cells, found outside the cords, into fetal Leydig cells, thus ultimately allowing the production of testosterone. Endothelial cells are associated to form the coelomic vessel, which promotes efficient export of testosterone into plasma.
The gene Sox9 is up-regulated immediately after Sry expression and is involved in the initiation and maintenance of Sertoli cell differentiation during the early phases of testis differentiation. The mechanism by which NR5A1 and SRY increase endogenous SOX9 expression was clearly demonstrated in human embryonal carcinoma cell line NT2/D1 (43).
Extracellular signaling pathways (Fgf9 and Igf1r/Irr/Ir) play a significant role in Sox9 expression. A model has been suggested in that the fate of the bipotential gonad is controlled by mutually antagonistic signals between Fgf9 andWnt4/Rspo1. In this model Sox9 up-regulates Fgf9-Fgfr2 and Fgf9 maintains Sox9 expression, forming a positive feed-forward loop in XY gonads. The balance between Fgf9 and Wnt4/Rspo1 signals is shifted in favor of Fgf9, establishing the male pathway. In addition, Sry inhibits β-catenin-mediated Wnt signaling (44). In the absence of this feed-forward loop between Sox9 and Fgf9, Wnt4/Rspo1, the activated β-catenin pathway, blocks Fgf9 and promotes the ovarian fate (45,46). Furthermore, Sox9 directly binds to the promoter of the Ptgds gene which encodes prostaglandin D synthase that mediates the production of PGD2 (47) which, in turn, promotes nuclear translocation of Sox9, facilitating Sertoli cell differentiation (48). Antagonism between Dmrt1 and Foxl2 comprises another step for sex-determining decision. Dmrt1 has been described as essential to maintain mammalian testis determination, preventing female reprogramming in the postnatal mammalian testis (49). MAP3K1 has been described to be important to the balance between SOX9/FGF9 to WNT/beta-catenin signaling in functional studies (50,51). However, the role of MAP3K1 in human sex-determination remains unknown as the downstream effectors of MAP3K1 in the human developing testis have not been identified (52). Similarly, the precise mechanism by which DHX37 interferes with testis determination/maintenance remains to be elucidated (53). Abnormalities in the expression (underexpression or overexpression or timing of expression) of genes involved in the cascade of testis determination can cause anomalies of gonadal development and consequently, 46,XY DSD. The absence, regression, or the presence of dysgenetic testes results in abnormal development of the genital ducts and/or external genitalia in thosepatients.
46,XY Gonadal Agenesis
Total absence of gonadal tissue confirmed by laparoscopy has rarely been described in XY subjects with female external and internal genitalia indicating the absence of testicular determination (54). Mendonca et al described a pair of siblings, one XY and the other XX, born to a consanguineous marriage, with normal female external and internal genitalia associated with gonadal agenesis (55). Pathogenic allelic variants in NR5A1 and LHX9 were later ruled out in these siblings (56). The origin of this disorder remains to be determined, but a defect in another gene essential for bipotential gonad development is the most likely cause of this disorder.
46,XY gonadal dysgenesis consists of a variety of clinical conditions, in which the development of the fetal gonad is abnormal and encompasses both a complete and a partial form. The complete form of gonadal dysgenesis was first described by Swyer et al. (57) and is characterized by female external and internal genitalia, lack of secondary sexual characteristics, normal or tall stature without somatic stigmata of Turner syndrome, eunuchoid habitus and the presence of bilateral dysgenetic gonads in XY subjects. Mild clitoromegaly is present in some cases.
The partial form of this syndrome is characterized by variable degrees of impaired testicular development and testicular function. These patients present a spectrum of atypical genitalia with or without Müllerian structures. Similar phenotypes can also result from a 45,X/46,XY karyotype.
Serum gonadotropin levels are elevated in both the complete and partial forms, mainly FSH levels, which predominate over LH levels. Testosterone levels are at the prepubertal range in the complete form. Meanwhile, in the partial form, it can range from prepubertal levels to normal adult male levels.
The clinical condition named embryonic testicular regression syndrome (ETRS) has been considered part of the clinical spectrum of partial 46,XY gonadal dysgenesis (58). In this syndrome, most of the patients present atypical genitalia or micropenis associated with complete regression of testicular tissue in one or both sides. Pathogenic/likely pathogenic variants in DHX37 were reported in patients with 46,XY GD at a frequency of 14%. Considering only the ETRS phenotype (micropenis and absence of uni- or bilateral testicular tissue), this frequency increases to 50% (53).The masculinization degrees of internal and external genitalia presented are related to the time and duration of the hormonal secretion, prior to cessation of testicular function. The dysgenetic testes showed disorganized seminiferous tubules and stroma with occasional primitive sex cords without germ cells (59). Familial cases of gonadal dysgenesis with variable degrees of genital atypia have been reported, and the nature of the underlying genetic defect is still unknown in several families, despite new genetic investigation methodologies available (58). Regarding the genetic etiology, 46,XY gonadal dysgenesis is heterogeneous and can result from defects of any gene involved in the process of gonadal formation.
The following review will focus on the main genes causing gonadal dysgenesis in humans, presenting as an isolated or syndromic phenotype.
Dysgenetic 46,XY DSD Due to Under Expression of GATA4 and FOG2/ ZFPM2 Genes
Gata4 (GATA-binding factor 4 gene) cooperatively interacts with several proteins to regulate the expression of genes involved in testis determination and differentiation as SRY, SOX9, NR5A1, AMH, DMRT1, STAR, CYP19A1, and others (60).
In humans, GATA4 variants were first described in patients with congenital heart defects without genital abnormalities (61). However, genitourinary anomalies, such as hypospadias and cryptorchidism, were described in 46,XY patients with deletion of the 8p23.1 region, in which GATA4 is located (62).
The p.G221R GATA4 pathogenic variant was identified in five members of a French family, three 46,XY DSD patients, two of them with cardiac anomalies, and in their apparently unaffected mothers (63).
The role of FOG2 in human testis development was corroborated by the identification of a balanced translocation (8;10) (q23.1;q21.1) in a patient with partial gonadal dysgenesis and congenital heart abnormalities (64). Bashamboo et al. identified missense FOG2 variants, using exome sequencing, in two patients with 46,XY gonadal dysgenesis. One patient carried the non-synonymous p.S402R heterozygous variant. The second patient carried the inherited homozygous p.M544I variant and the de novo heterozygous p.R260Q variant. The p.M544I variant by itself has little effect on the biological activity of FOG2 protein in transactivation of the gonadal promoters, but it shows reduced binding with GATA4. In the in vitro assays, a combination of both the p.R260Q and the p.M544I variants altered the biological activity of the FOG2 protein on specific downstream targets, as well as obliterated its interaction with GATA4. In the patient, the two variants together may result in an imbalance of the delicate equilibrium between antagonistic male and female pathways leading ultimately to gonadal dysgenesis (65). Although several GATA4 and FOG2/ZFPM2 variants have been identified in 46,XY DSD patients, the real role of the majority of them in the etiology of gonadal disease is still unclear. The re-study of seven GATA4 and ten FOG2/ZFPM2 variants previously identified by Eggers et al. (26) in a cohort of 46,XY DSD patients, using updated tools and testing their molecular activity in the context of gonadal signaling by in vitro assays, support that the majority of them are benign in their contribution to 46,XY DSD. Only one variant (p. W228C) located in the conserved N-terminal zinc finger of GATA4, was considered pathogenic, with functional analysis confirming differences in its ability to regulate Sox9 and AMH, and in protein interaction with ZFPM2 (66).
Dysgenetic 46,XY DSD Due to Under Expression of the CBX2 gene
In humans, variants in both CBX2.1 and CBX2.2 isoforms were associated with 46,XY DSD (67,68).
The compound heterozygous CBX2.1 variants, c.C293T (p.P98L) and c.G1370C (p.R443P), inherited from the father and the mother respectively, was identified in a 46,XY patient, who was born with a completely normal female phenotype. The patient had uterus and histologically normal ovaries (67) and high serum FSH levels. Her phenotype resembles the Cbx2 knock-out XY mice phenotype (41). Cbx2 (M33) knockout mice present hypoplastic gonads in both sexes, but a small or absent ovaries are observed in the XY Cbx2 knockout, consequently to the reduced expression of Sry and Sox9 in the gonadal tissue (41). Functional studies demonstrated that these variants do not bind to, or adequately regulate the expression of target genes important for gonadal development, such as NR5A1 (67).
Mutated CBX2.2 isoforms were also implicated in the etiology of partial 46,XY gonadal dysgenesis in two other patients. Each patient carried a distinct variant, the p. C132R (c.394T>C) and the C154fs (c.460delT). These CBX2.2variants were shown to be related to a defective expression of EMX2 in the developing gonad (68). However, analysis of populational genetics data indicates that p.C154fs is present in general populations at high frequency, inconsistent with causing gonadal dysgenesis (69).
46,XY DSD Due to Under Expression of the WT1 Gene
The Wilms’ tumor suppressor gene (WT1) is located on 11p13, and encodes a zinc-finger transcription factor involved in the development and function of the kidneys and gonads. The WT1 contains 10 exons, of which exons 1–6 encode a proline/glutamine-rich transcriptional-regulation region and exons 7–10 encode the four zinc fingers of the DNA-binding domain. Four major species of RNA with conserved relative amounts, different binding specificities, and different subnuclear localizations are generated by two alternative splicing regions (70). Splicing at the first site results in either inclusion or exclusion of exon 5. The second alternative splicing site is in the 3’ end of exon 9 and allows the inclusion or exclusion of three amino acids lysine, threonine and serine between the third and fourth zinc fingers, resulting in either KTS-positive or negative isoforms. Isoforms that only differ by the presence or absence of the KTS amino acids have different affinities for DNA and, therefore, possibly different regulatory functions (70). A precise balance between WT1 isoforms is necessary for its normal function (71).
WT1 presents a complex network of interaction with several protein systems so that abnormalities in it can determine a wide phenotypic spectrum in XY and XX individuals (72). Several syndromes are associated with WT1 pathogenic variants, including: WAGR, Denys-Drash and Frasier syndrome.
WAGR syndrome is characterized by Wilms’ tumor, aniridia, genitourinary abnormalities and mental retardation. Genitourinary anomalies are frequently observed, such as renal agenesis or horseshoe kidney, urethral atresia, hypospadias, cryptorchidism and more rarely atypical genitalia (73). Heterozygous deletions of WT1 and othercontiguous genes are the cause of this syndrome (74). Deletions of PAX6 gene are related to the presence of aniridia in these patients. Severe obesity is present in a substantial proportion of subjects with the WAGR syndrome, and the acronym WAGRO has been suggested for this condition (75). The phenotype of obesity and hyperphagia in WAGRO syndrome is attributable to deletions that determine haploinsufficiency of the BDNF gene (Brain-Derived Neurotrophic Factor) (76).
Le Caignec et al. described a 46,XY patient with an interstitial deletion of approximately 10 Mb located on 11p13, encompassing WT1 and PAX6 , who presented a female external and internal genitalia, an unusual phenotype of WAGR syndrome (77). This report demonstrated an overlap of clinical and molecular features in WAGR, Frasier and Denys-Drash syndromes that confirms these conditions as a spectrum of disease due to WT1 alterations.
Denys-Drash syndrome is characterized by dysgenetic 46,XY DSD associated with early-onset renal failure (steroid-resistant nephrotic syndrome with diffuse mesangial sclerosis and progression to end-stage kidney disease) and Wilms´ tumor development in the first decade of life (78). Müllerian duct differentiation varies according to the Sertoli cells' function. The molecular defect of this syndrome is the presence of heterozygous missense allelic variants in the zinc finger encoding exons (DNA-binding domain) of WT1 gene (79). Gonadal development is impaired to variable degrees, resulting in a spectrum of 46,XY DSD (80).
Frasier syndrome is characterized by a female to atypical external genitalia phenotype in 46,XY patients, streak or dysgenetic gonads, which are at high risk for gonadoblastoma development, and renal failure (early steroid-resistant nephrotic syndrome with focal and segmental glomerular sclerosis). In these patients the progression to end-stage renal disease often occurs in adolescence, although the late-onset nephropathy has also been described in Frasier syndrome (81), reinforcing that patients carrying WT1 pathogenic variants should have the renal function carefully monitored (82).
Constitutional heterozygous variants of the WT1, almost all located at intron 9, are found in patients with Frasier syndrome, leading to a change in splicing that results in reversal of the normal KTS positive/negative ratio from 2:1 to 1:2 (78,83). Exonic variants have been also associated with Frasier syndrome (84).
The report of atypical external genitalia (84) , the presence of Wilms’ tumor (85), and the description of exonic variants in the DNA binding domain of WT1 gene (84) in patients with Frasier syndrome indicate an overlap of clinical and molecular features between Denys Drash and Frasier syndromes.
46,XY DSD Due to Under Expression of the NR5A1/SF1 Gene
NR5A1 was originally identified as a master-regulator of steroidogenic enzymes in the early 1990s following the Keith L. Parker and Kenichirou Morohashi inspiring work (86-88). NR5A1 has since been shown to control many aspects of adrenal and gonadal function (37,89), NR5A1, together with several signaling molecules, are also involved in adrenal stem cell maintenance, proliferation and differentiation inducing adrenal zonation, probably acting in the progenitor cells (90).
Homozygous 46,XY null mice (−/−) have adrenal agenesis, complete testicular dysgenesis, persistent Müllerian structures, partial hypogonadotropic hypogonadism, and other features such as late-onset obesity (91). Therefore, it was clear demonstrated that Nr5a1 is an essential factor in sexual and adrenal differentiation, and a key regulator of adrenal and gonadal steroidogenesis and also of the hypothalamic-pituitary-gonadal axis.
The first reported human case of NR5A1 pathogenic variant, the heterozygous p.G35E, was a 46,XY patient who presented female external genitalia and Müllerian duct derivatives, indicating the absence of male gonadal development, associated with adrenal insufficiency. This patient presented with salt-losing adrenal failure in early infancy and was thought to have a high block in steroidogenesis (e.g., in CYP11A1, STAR) affecting both adrenal and testicular functions. However, the identification of streak-like gonad and Müllerian structures was consistent with testicular dysgenesis, thereby, a disruption of a common developmental regulator such as NR5A1 was hypothesized. The patient was found to have a de novo heterozygous p.G35E change in the P-bo of NR5A1, which is important in dictating DNA binding specificity through its interaction with DNA response elements in the regulatory regions of target genes (92).
The second report of NR5A1 defects in humans was described by Biason-Lauber and Schoenle, in a 14-month-old 46,XX girl who had presented with primary adrenal insufficiency and seizures (93) . She had a de novo heterozygous NR5A1 change resulting in the p.R255L variant into the proximal part of the ligand-like binding domain of the protein. The ovaries were detected by MRI scan and Inhibin A levels were normal for her age, suggesting that NR5A1 change had not disrupted ovarian function. The follow up of this girl until 16.5 years old showed a normal puberty and regular menstruation showing that phenotypic variant of NR5A1 allelic variant in a 46, XX affected person includes adrenocortical insufficiency but no ovarian dysfunction at pubertal age (94).
The third report of NR5A1 defects in humans was found in an infant with a similar phenotype of the first case: primary adrenal failure and 46,XY DSD. However, this child had inherited the homozygous p.R92Q alteration in a recessive manner (95). The change lies within the A-box of NR5A1, which interferes with monomeric DNA binding stability, but in vitro functional activity was in the order of 30–40% of the wild type (95-97). Carrier parents showed normal adrenal function suggesting that the loss of both alleles is required for the phenotype development when disrupted protein keeps this level of functional activity. In addition, another family has been reported with a homozygous missense variant (p.D293N) in the LBD of NR5A1 (98). This change also showed partial loss-of-function (50%) in gene transcription assays.
In 2004, we reported the fourth NR5A1 deleterious variant in humans, which brought two novel variables to the NR5A1 phenotype: it was the first frameshift variant and it was identified in a 34-year-old 46,XY DSD female with normal adrenal function (99). Another interesting aspect in this patient was the absence of gonadal tissue at laparoscopy. Since she had atypical genitalia and absence of Müllerian derivatives, we assumed that testicular tissue regressed completely late in fetal life.
NR5A1 changes associated with 46,XY DSD are usually frameshift, nonsense or missense changes that affect DNA-binding and gene transcription (96). Most of the point variants identified in NR5A1 are located in the DNA-binding domain of the protein. The p.L437Q variant, the first located in the ligand-binding region, was identified in a patient with a mild phenotype, a penoscrotal hypospadias. This protein retained partial function in several NR5A1-expressing cell lines and its location points to the existence of a ligand for NR5A1, considered an orphan receptor so far (97). NR5A1 is bound to sphingosine (SPH) and lyso-sphingomyelin (lysoSM) under basal conditions (100,101).
Progressive androgen production and virilization in adolescence has been observed in several XY patients with NR5A1 variants, in contrast to the severe under virilized external genitalia found in most patients (101,102). The almost normal testosterone levels after hCG stimulation test or at pubertal age suggest that NR5A1 action might be less implicated in pubertal steroidogenesis than during fetal life.
In contrast, fetal Sertoli cell function seems to be preserved in most patients with heterozygous NR5A1 variants based on the common observation of absent Müllerian derivatives and primitive seminiferous tubules in histology. The reviewed data of seventy-two 46,XY DSD patients with NR5A1 pathogenic variants reported in the literature, for whom information on the presence or absence of Müllerian derivatives was available, suggested that Müllerian derivatives are present in about 24% of the cases. However, persistently elevated FSH levels after puberty found in all patients studied suggest an impairment of Sertoli cells function in post pubertal age.
More than 180 different NR5A1 variants, distributed across the full length of the protein, have been described and the majority are nonsynonymous variants (103-105).
Most of these variants are in the DNA binding domain and are in heterozygous state or compound heterozygous state with the p.G46A (rs1110061) variant. A clear correlation between the location of a variant, it’s in vitro functional performance and the associated phenotype is not observed. Indeed, family members bearing the same NR5A1variant may present with different phenotypes (106).
The contribution of other genetic modifiers has been suggested to explain phenotypic variability. Exome sequencing analyses of DSD patients have identified pathogenic variants or variants of uncertain significance in several genes involved in sexual development (42). In a 46,XY patient with atypical external genitalia, palpable inguinal gonads, absent uterus in pelvic ultrasonography and poor testosterone response to hCG stimulation, Mazen and colleagues identified, by exome sequencing, the previously described p.Arg313Cys NR5A1 variant in compound heterozygous state with a p.Gln237Arg MAP3K1 variant 27169744 (107). This NR5A1 variant was previously reported in association with mild hypospadias (108), and a possible digenic inheritance was proposed to explain the phenotypic heterogeneity (107).
In several cohort studies, NR5A1 changes have been reported in approximately 10–15% of the individuals with gonadal dysgenesis (89,96). Although many of the heterozygous changes are de novo, about one-third of these changes have been shown to be inherited from the mother in a sex-limited dominant manner (96). These women are at potential risk of primary ovarian insufficiency but while fertile they can pass NR5A1 heterozygous changes to their children. This mode of transmission can mimic X-linked inheritance (96). The features in different affected family members can be variable.
A different role of NR5A1 in human reproductive function was described by Bashamboo and co-workers (109). They investigated whether changes in NR5A1 could be found in a cohort of 315 men with normal external genitalia and non-obstructive male factor infertility where the underlying cause was unknown (109). Analysis of NR5A1 in this cohort identified heterozygous changes in seven individuals; all of them were located within the hinge region of the NR5A1 protein. The men who harbored NR5A1 changes had more severe forms of infertility (azoospermia, severe oligozoospermia) and in several cases low testosterone and elevated gonadotropins were found. A serial decrease in sperm count was found in one-studied men, raising the possibility that heterozygous changes in NR5A1 might be transmitted to offspring, especially if fatherhood occurs in young adulthood rather than later in life (110). As progressive gonadal dysgenesis is likely, gonadal function should be monitored in adolescence and adulthood, and early sperm cryopreservation considered in male patients, if possible. In conclusion, this study shows that changes in NR5A1 may be found in a small subset of phenotypically normal men with non- obstructive male factor infertility where the cause is currently unknown. These individuals may be at risk of low testosterone in adult life and may represent part of the adult testicular dysgenesis syndrome (110,111).
A novel heterozygous missense variant (p.V355M) in NR5A1 was identified in one boy with a micropenis and testicular regression syndrome (112). NR5A1 variants have also been identified in familial and sporadic forms of 46,XX primary ovarian insufficiency not associated with adrenal failure (98,113). Most of these women harbored heterozygous alterations in NR5A1 and had been identified in families with histories of 46,XY DSD and 46,XX POI. Heterozygous NR5A1 changes were also found in two girls with sporadic forms of POI (98). In one large kindred, a partial loss-of-function NR5A1 change (p.D293N) was inherited in an autosomal recessive manner. These 46,XX women with p.D293N NR5A1 variant presented with either primary or secondary amenorrhea and with a variable age of features onset. The detection of NR5A1 alterations in 46,XX ovarian failure shows that NR5A1 is also a key factor in ovarian development and function in humans. Thus, some 46,XX women with NR5A1 variants have normal ovarian function and can transmit the variant in a sex-limited dominant pattern. Therefore, the inheritance patterns associated with NR5A1 changes can be autosomal dominant, autosomal recessive or sex-limited dominant.
NR5A1 defects can be found in association with a wide range of human reproductive phenotypes such as 46,XY and 46,XX disorders of sex development (DSD) associated or not with primary adrenal insufficiency, male infertility, primary ovarian insufficiency and finally testicular or ovo-testicular 46,XX DSD (101) (103) (Table 4). Spleen development anomalies have been described in patients with NR5A1 variants (103).
Table 2. Spectrum of Phenotypes Caused by NR5A1 Defects
|
Karyotype
|
Phenotypes
|
Number of reported patients
|
Reference
|
46,XY
|
DSD and adrenal insufficiency
|
2
|
(92,95)
|
DSD without adrenal insufficiency
|
69
|
(63,89,96,98,100,101,103,106)
|
Male infertility
|
10
|
(63)
|
Ovotesticular DSD and genitopatellar syndrome*
|
1
|
(114)
|
46,XX
|
Adrenal insufficiency
|
2
|
(92,93)
|
Female infertility, POI
|
14
|
(98,101,103)
|
(Ovo) testicular DSD
without adrenal insufficiency
|
11
|
(98,115)
|
46,XY DSD Due to Under Expression of the SRY Gene
Most of the authors reported pathogenic allelic variants in SRY gene in less than 20% of the patients with complete 46,XY gonadal dysgenesis (116-118). In the partial form, the frequency of SRY variants is even lower than in the complete form. To date, most of the SRY variants are located in the HMG box, showing the critical role of this domain, and are predominantly de novo variants. However, some cases of fertile fathers and their XY affected children, sharing the same altered SRY sequence, have been reported (116,119). In a few of these cases, the father’s somatic mosaicism for the normal and mutant SRY gene has been proven (120) The variable penetrance of SRY variants in familial cases have been described in SRY mutant proteins with relatively well preserved in vitro activity (121).
Dysgenetic 46,XY DSD Associated with Campomelic Dysplasia (Under Expression of the SOX9 Gene)
SRY-related HMG-box gene 9 (SOX9) is a transcription factor involved in chondrogenesis and sex determination. SOX9 gene, located on human chromosome 17, is a highly conserved HMG family member and it is also implicated in the male sex-determining pathway (122,123).
Pathogenic allelic variants in SOX9 have been identified in heterozygous state in patients with Campomelic dysplasia (122). This syndrome is characterized by severe skeletal malformations associated with dysgenetic 46,XY DSD. These patients have variable external genitalia ranging from that of normal male with cryptorchidism to atypical or female genitalia, and the internal genitalia may include vagina, uterus, and fallopian tubes (124).
Intact SOX9 were also reported in patients with Campomelic dysplasia and 46,XY gonadal dysgenesis. The genomic analysis of the SOX9 locus in these patients identified a key regulatory element termed RevSex, located approximately 600 kb upstream from SOX9. RevSex is duplicated in individuals with 46,XX (ovo)testicular DSD and deleted in individuals with 46,XY GD (125,126). Moreover, structural changes involving multiple regions both upstream and downstream of the SOX9 gene have been associated with non-syndromic XY DSD (127,128). These findings indicate that variants located in the regulatory elements of SOX9 should be routinely screened in a DSD diagnostic setting (69).
Dysgenetic 46,XY DSD Due to Under Expression of the FGF9/FGFR2 Genes
The importance of Fgf9/Fgfr2 signaling pathway in mouse testis determination is well known (129,130). In the developing testis occurs a positive feedback loop among Fgf9/Fgfr2/Sox9; Fgf9 is upregulated by Sox9 and signals through Fgfr2 maintain Sox9 expression (129) and this loop represses Wnt4 (131).
Mice homozygous for a null variant in Fgf9 or Fgfr2 exhibit male-to-female sex reversal, with all testis-specific cellular events being disrupted, including cell proliferation, mesonephric cell migration, Sertoli cell differentiation, and testis cord formation (129,130,132). However, in human sex development the role of FGF9 and FGFR2 remains unclear.
In humans, the only reported pathogenic variants in FGF9 are associated with craniosynostosis or multiple synostosis phenotypes, and no FGF9 variants were identified in 46,XY GD patients (133).
Human FGFR2 variants have been related with some syndromes as lacrimo-auriculo-dento-digital, characterized by tear tract, ear, teeth and digit abnormalities (133) and craniosynostosis syndromes including Crouzon, Pfeiffer, Apert and Antley-Bixler syndromes (134-136). FGFR2 variants can lead to loss (LAAD syndrome) or gain (craniosynostosis syndromes) of function in these disorders (137). No gonadal defects were described in patients with LADD or craniosynostosis syndromes.
A single 46,XY patient with gonadal dysgenesis and craniosynostosis was described by Bagheri-Fam et al (138). This patient had abnormalities which are identified in several craniosynostosis syndromes (short stature, brachycephaly, proptosis, down slanting palpebral fissures, low-set dorsally rotated ears, reduced extension at the elbows but absence of hand and feet anomalies). She also presented female external genitalia, primary amenorrhea and gonadal dysgenesis with dysgerminoma. DNA sequencing revealed a cysteine-to-serine substitution at position 342 in the FGFR2c isoform (p.C342S). Cys342 substitutions by Ser or other amino acids (Arg/Phe/Trp/Tyr) occur frequently in the craniosynostosis syndromes Crouzon and Pfeiffer but these patients do not present gonadal abnormalities. Variants in the 2c isoform of FGFR2 is in agreement with knockout data showing that FGFR2c is the critical isoform during sex determination in the mouse. Taken together, these data suggest that the FGFR2c c.1025G>C (p.C342S) variant might contribute to 46,XY DSD in this patient. The authors proposed that this heterozygous variant leads to gain of function in the skull, but to loss of function in the developing gonads and that she might harbor a unique set of modifier genes, which exacerbate this testicular phenotype (138).
The authors proposed that the p.C342S heterozygous variant in FGFR2c leads to gain of function in the skull, but loss of function in the developing gonads; and that the presence of modifier genes would exacerbate the testicular phenotype in this patient (138). However, the presence of a pathogenic variant involving other DSD genes, cannot be completely excluded in this patient.
Dysgenetic 46,XY DSD Due to Disruption in Hedgehog Signaling
DESERT HEDGEHOG (DHH) GENE
It is a member of the hedgehog family of signaling proteins, is located in chromosome 12-q13.1 and is one of the genes involved in the testis-determining pathway (139). Dhh seems to be necessary for Nr5a1 up-regulation in Leydig cells in mice (140). To date, six homozygous variants have been described in DHH gene in 46,XY patients conferring phenotypes ranging from partial to complete gonadal dysgenesis, associated or not with polyneuropathy. The first one, the homozygous missense variant (p.M1T) is located at the initiation codon of exon 1 and was found in a 46,XY patient with partial gonadal dysgenesis associated with polyneuropathy (141). Two other variants, one the p.L162P located at exon 2 and the other the p.L363CfsX4 located in exon 3 were identified in three patients with complete gonadal dysgenesis without polyneuropathy; two of them harbored gonadal tumors (bilateral gonadoblastoma and dysgerminoma, respectively) (142). Later, the c.1086delG variant was identified in heterozygous state in two patients with partial gonadal dysgenesis (143). In addition, two novel homozygous variants were described in two patients with complete 46,XY gonadal dysgenesis without clinically overt polyneuropathy (144). In both sisters, clinical neurological examination revealed signs of a glove and stocking like polyneuropathy. The first defect, the c.271_273delGAC resulted in deletion of one amino acid (p.D90del) and the second one, a duplication c.57_60dupAGCC resulted in a premature termination of DHH protein (144) . The p.R124Q variant was identified by exome sequencing in two sisters of a consanguineous family with 46, XY gonadal dysgenesis and testicular seminoma (145).
HEDGEHOG ACETYL-TRANSFERASE (HHAT) GENE
The HHAT protein is a member of the MBOAT family of membrane-bound acyl-transferases which catalyzes amino-terminal palmitoylation of Hh proteins. The novel variant (p.G287V) in the HHAT gene was found in a syndromic 46,XY DSD patient with complete gonadal dysgenesis and skeletal malformation by exome sequencing. This variant disrupted the ability of HHAT protein to palmitoylated Hh proteins including DHH and SHH (146) In mice, the absence of Hhat in the XY gonad did not affect testis-determination, but impaired fetal Leydig cells and testis cords development (146). The phenotype of the girl carrying the homozygous p.G287V variant is a rare combination of gonadal dysgenesis and chondrodysplasia. Moreover, a de novo dominant variant in the MBOAT domain of HHAT was reported in association with intellectual disability and apparently normal testis development (147).
46,XY DSD Due to Under Expression of the DMRT1 Gene
Raymond et al identified both DNA-binding Motif (DM) domain genes expressed in testis (DMRT1 and DMRT2) located in chromosome 9p24.3, a region associated with gonadal dysgenesis and 46,XY DSD (148-150). The human 9p monosomy syndrome is characterized by variable degrees of 46,XY DSD, from female genitalia to male external genitalia with cryptorchidism associated to agonadism, streak gonads or hypoplastic testes and internal genitalia disclosing normal Müllerian or Wolffian ducts, mental retardation and craniofacial abnormalities (151). Gonadal function varies from insufficient to near normal testicular production. It is inferred that haploinsufficiency of DMRT1and DMRT2 primarily impairs the formation of the undifferentiated gonad, leading to various degrees of testis or ovary formation defects (151).
Although 9p24 deletions are a relatively common cause of syndromic 46,XY gonadal dysgenesis, the pathogenic variants within DMRT1 are rarely identified (152).
Genomic–wide copy number variation screening revealed that DMRT1 deletions were associated with isolated 46,XY gonadal dysgenesis in addition to inactivation variants (133,148). In vitro studies to analyze the functional activity of the DMRT1 (p.R111G) variant identified by exome sequencing in a patient with 46,XY complete gonadal dysgenesis, indicated that this protein had reduced DNA affinity and altered sequence specificity. This mutant DMRT1, when mixed with the wild-type protein bound as a tetramer complex to an in vitro Sox9 DMRT1-binding site, differs from the wild-type DMRT1 that is usually bound as a trimer. This suggests that a combination of haploinsufficiency and a dominant disruption of the normal DMRT1 target binding site is the cause of the abnormal process of testis-determination seen in this patient (153).
Matson et al. (2011) have shown in mice that Dmrt1 and Foxl2 create another regulatory network necessary for maintenance of the testis during adulthood. Loss of Dmrt1 in mouse Sertoli cells induces the reprogramming of those into granulosa cells, due to Foxl2 upregulation. Consequently, theca cells are formed, estrogens are produced, and germ cells appear feminized (49).
ATR-X Syndrome (X-linked α-Thalassemia and Mental Retardation)
ATR-X syndrome results from variants in the gene that encodes for X-linked helicase-2, implicating ATR-X in the development of the human testis (154). Genital anomalies leading to a female sex of rearing were reported in several affected 46,XY patients with ATR-X syndrome (155).
ATR-X syndrome is characterized by severe mental retardation, alpha thalassemia and a range of genital abnormalities in 80% of cases (154). In addition to these definitive phenotypes, patients also present with typical facial anomalies comprising a carp-like mouth and a small triangular nose, skeletal deformities and a range of lung, kidney, and digestive problems. A variety of phenotypically overlapping conditions (Carpenter-Waziri syndrome, Holmes-Gang syndrome, Jubert-Marsidi syndrome, Smith-Fineman-Myers syndrome, Chudley-Lowry syndrome and X-linked mental retardation with spastic paraplegia without thalassemia) have also been associated with ATRX variants (154).
ATRX lies on the X chromosome (Xq13) and the disease has been confined to males; in female carriers of an ATRX variant, the X-inactivating pattern is skewed against the X chromosome carrying the mutant allele.
Urogenital abnormalities associated with variants in human ATRX range from undescended testes to testicular dysgenesis with female or atypical genitalia. Duplication of Xq12.2-Xq21.31 that encompasses ATRX along with other genes has been described in a male patient with bilateral cryptorchidism and severe mental retardation. The patient entered spontaneous puberty by the age of 12 and developed bilateral gynecomastia (156). There are two major functional domains in ATRX protein: 1- the ATRX-DNMT3-DNMT3L (ADD) domain at the N-terminus and 2- the helicase/ATPase domain at the C-terminal half of the protein, both acting as chromatin remodeling. variants in the ADD domain have been related to severe psychomotor impairment associated with urogenital abnormalities. On the other hand, variants in the C-terminus region have been related with mild psychomotor impairment without severe urogenital abnormalities (157,158).
Although all cases of severe genital abnormality reported in ATRX syndrome have been associated with severe mental retardation, this is not true for alpha-thalassemia. The role of ATRX in the sexual development cascade is poorly understood and it is suggested that it could be involved in the development of the Leydig cells (159).
Dysgenetic 46,XY DSD Due to Under Expression of the MAP3K1 Gene
MAPK signaling pathway role in mammalian sex-determination is still poorly understood. In mice, it has been shown that the Map3k4 gene is essential for testicular determination, since the lack of activity of this protein leads to failure of testicular cord development and disorganization of gonadal tissue in development (160). In mice, the reduction of the Gadd45/Map3k4/p38 pathway activity is associated with a reduction in the Sry expression in the XY mice gonad at sex-determination causing sex-reversal in these animals (161). Studies with knock-in animals for the Map3k1 gene demonstrated a lower repercussion in the testicular tissue, which present a reduction in the Leydig cells number (162,163). However, in patients with 46, XY gonadal dysgenesis, different non-synonymous allelic variants were identified in the MAP3K1 gene. The first variant described was identified for mapping by linkage analysis of an autosomal sex-determining gene locus at the long arm of chromosome 5 in two families with 46,XY DSD, including patients with complete and partial gonadal dysgenesis. The splice-acceptor variant c.634-8T>A in the MAP3K1 disrupted RNA splicing and was segregated with the phenotype in the first family. Variants in the MAP3K1 were also demonstrated in the second family (p.G616R) and in two of 11 sporadic 46,XY DSD patients (p.L189P, p.L189R) studied (51,164). Subsequently, the two novel variants p.P153L and c.2180- 2A>G in the MAP3K1 were identified in non-syndromic patients with 46,XY gonadal dysgenesis. Functional studies of mutated MAP3K1 proteins identified change in phosphorylation targets in subsequent steps of the cascade of MAP3K1, p38 and ERK1/2 and enhanced the binding of the Ras homolog gene family, member A (RHOA) to the MAP3K1 complex (51). In normal male gonadal development, the binding of MAP3K1 to the RHOA protein promotes a normal phosphorylation of p38 and ERK1/2, and a blockade of the β-catenin pathway is determined by MAP3K4. In the female development, hyperphosphorylation of p38 and ERK1/2 occurs and the presence of p38 and ERK1/2 hyperphosphorylated determine the activation of the β-catenin pathway, that result in a block of the positive feedback pathway of SOX9 and the testicular development (51) .
Cohorts of patients with 46,XY DSD studied by a targeted gene panel have found several new potentially deleterious variants and uncertain significance variants in the MAP3K1 (26). Although the findings strongly indicate the participation of the MAP3K1 variants in the etiology of testicular development abnormalities, a better understanding of the mechanisms of MAPK pathway in the gene regulatory networks of the human testicular determination process is still necessary (52,107).
46,XY DSD Due to Over Expression of the NR0B1/DAX1 Gene
Male patients with female or atypical external and internal genitalia due to partial duplications of Xp in the presence of an intact SRY gene have been described (28). These patients present with dysgenetic or absent gonads associated or not with mental retardation, cleft palate, and dysmorphic face. Bardoni et al. identified in these patients, a common 160-kb region of Xp2 containing NR0B1/DAX1 gene named dosage sensitive sex locus which, when duplicated, resulted in 46,XY DSD (164).
The large duplications of Xp21 reported prior to array-CGH and MLPA techniques were identified by conventional karyotyping. Patients carried large genomic rearrangements involving several genes. In these patients, the presence of XY gonadal dysgenesis was part of a more complex phenotype, which also included dysmorphic features and/or mental retardation (165).
Interestingly, in all cases with isolated 46,XY gonadal dysgenesis, the IL1RAPL1 gene located immediately to the duplication containing NR0B1/DAX1, is not disrupted. Deletions or variants of this gene have been identified in patients with mental retardation (166). Disruption of this gene could explain the mental retardation previously described in patients with larger Xp21 duplications (167).
Several patients with isolated 46,XY gonadal dysgenesis due to duplications of Xp21 have been described. The first report identified a 637 kb tandem duplication on Xp21.2 that in addition to NR0B1/DAX1 includes the four MAGEBgenes in two sisters with isolated 46,XY gonadal dysgenesis and gonadoblastoma (168). The second case exhibited a duplication with approximately 800 kb in size and, in addition to NR0B1/DAX1, contains the four MAGEB, Cxorf21 and GK genes. The healthy mother was a carrier of the duplication (169).
Smyk et al. described a 21-years-old 46,XY patient manifesting primary amenorrhea, a small immature uterus, gonadal dysgenesis and absence of adrenal insufficiency with a submicroscopic deletion (257 kb) upstream of NR0B1/DAX1. The authors hypothesized that loss of regulatory sequences may have resulted in up-regulation of DAX1 expression, consistent with phenotypic consequences of NR0B1/DAX1 duplication (170).
By using array-CGH and MLPA techniques, additional NR0B1/DAX1 locus duplications have been identified in patients with isolated 46,XY gonadal dysgenesis (28,169,171).
Barbaro et al. identified a relatively small NR0B1/DAX1 locus duplication responsible for isolated complete 46,XY gonadal dysgenesis in a large English family (28). The duplication extends from the MAGEB genes to part of the MAP3K7IP3 gene, including NR0B1, CXorf21, and GK genes. Unfortunately, the authors were unable to set up the rearrangement mechanism and distinguish between a nonallelic homologous recombination or a nonhomologous end joining mechanism. Therefore, until now, there is not a direct proof that an isolated NR0B1/DAX1 duplication is sufficient to cause 46,XY gonadal dysgenesis in humans, suggesting that other contiguous genes located in the DSS locus, should be involved in dosage-sensitive 46,XY DSD.
X-inactivation patterns in fertile female carriers of each of the three small NR0B1 locus duplications were analyzed (169). They established that female carrier of macroscopic Xp21 duplications are healthy and fertile due to the preferential inactivating of the duplicated chromosome and thereby protecting them from increased NR0B1 expression (169).
46,XY DSD Due to the Over Expression of WNT4 Gene
The WNT4 (wingless-type mouse mammary tumor virus integration site member 4) gene belongs to a family that consists of structurally related genes that encode cysteine-rich secreted glycoproteins that act as extracellular signaling factors (172).
Overexpression of the WNT4 and RSPO1 may be a cause of 46,XY DSD. A 46,XY newborn infant, with multiple congenital anomalies including bilateral cleft lips and palate, intrauterine growth retardation, microcephaly, tetralogy of Fallot, atypical external and internal genitalia, and undescended gonads consisted of rete testes and rudimentary seminiferous tubules, who carried a duplication of 1p31-p35, including both WNT4 and RSPO1 genes, was reported (173). In vitro functional studies showed that Wnt4 up-regulates Nr0b1/Dax1 in Sertoli cells, suggesting that Nr0b1/Dax1 overexpression was the cause of 46,XY DSD in this infant (174).
Table 3. Phenotypic Spectrum of Defects in the Genes Involved in Human Male Sex Determination
|
Genes
|
Chromosome position
|
Molecular
defect
|
External
genitalia
|
Müllerian ducts derivatives
|
Testes
|
Associated anomalies
|
Associated Syndrome
|
ARX
|
Xp22
|
Deletion/ Inactivating variants
|
Atypical/ micropenis with cryptorchidism
|
-
|
Dysgenetic
|
Abnormal psychomotor development, epilepsy, spasticity, and intellectual disability
|
X-linked lissencephaly, Proud syndrome,
Ohtahara syndrome
|
ATRX
|
Xq13
|
Inactivating variants
|
Atypical / Male with cryptorchidism
|
-
|
Dysgenetic
|
Severe psycho-motor retardation, dysmorphic face, cardiac and skeletal abnormalities, thalassemia
|
Alpha thalassemia and mental retardation X-linked
|
CBX2
|
17q25
|
Inactivating variants
|
Female
|
+
|
Normal
Ovary
|
No
|
No
|
DHH
|
12q12
|
Inactivating variants
|
Female/Atypical
|
+/-
|
Dysgenetic / Testis
|
Minifascicular
neuropathy
|
No
|
DHX37
|
12q24.31
|
Inactivating variants
|
Male with cryptorchidism and micropenis, Atypical
|
+/-
|
Dysgenetic/
Absent
|
No
|
No
|
DMRT1
|
9p24
|
Deletion/Inactivating variants
|
Female/ Atypical/ Male with cryptorchidism
|
+/-
|
Dysgenetic/Absent/ Hypoplastic
|
Craniofacial Abnormalities, microcephaly, mental retardation
|
No
|
DSS locus
(DAX-1 /MAGEB)
|
Xp21
|
Gene
duplication
|
Female/ Atypical/ male
|
+/-
|
Dysgenetic/
Absent
|
Mental retardation, cleft palate, dysmorphic face
|
No
|
FGFR2
|
10q26
|
Inactivating variants
|
Female
|
ND
|
Dysgenetic
|
Short stature, craniofacial abnormalities, elbow and knee contractures
|
Craniosynostosis
syndrome
|
FOG2/ZFPM2
|
8q23
|
Balanced translocation, inactivating variants
|
Male
|
-
|
Probable
dysgenetic
|
Heart defects
|
No
|
GATA4
|
8p23
|
Inactivating variants
|
Atypical / male with micropenis
|
-
|
Normal/
Dysgenetic
|
Heart defects
|
No
|
HHAT
|
1q32
|
Inactivating variants
|
Female
|
+
|
Dysgenetic
|
Chondrodysplasia
|
Nivelon-Nivelon-Mabille syndrome
|
MAP3K1
|
5q11.2
|
Inactivating mutation
|
Female/Atypical
|
+
|
Dysgenetic
|
No
|
No
|
MYRF
|
11q12.2
|
Inactivating variants
|
Female/Atypical
|
-
|
ND
|
Congenital heart defects, urogenital anomalies, congenital diaphragmatic hernia, and pulmonary hypoplasia
|
Cardiac urogenital syndrome
|
NR5A1
|
9q33
|
Inactivating variants
|
Female/Atypical/ Male with cryptorchidism
Male with spermatogenic failure
|
+/-
|
Normal/
Dysgenetic/
Absent
|
Adrenal
Insufficiency
|
No
|
PPP1R12A
|
12q21.2- q21.31
|
Inactivating variants
|
Female/Atypical
|
+/-
|
Dysgenetic
|
Genitourinary and/or brain malformations
|
No
|
SOX9
|
17q24.3-25.1
|
Inactivating variants,
5’ and 3’ Rearrangements
|
Female/ Atypical Male
|
+/-
|
Dysgenetic
|
Severe skeletal defects
|
Campomelic
displasia
|
SRY
|
Yp11.3
|
Inactivating variants
|
Female/ Atypical
|
+
|
Dysgenetic
|
No
|
No
|
WNT4
/RSPO1 locus
|
1p34.3-p35
|
Gene duplication
|
Atypical
|
+
|
Dysgenetic
|
Cleft lips and palate, tetralogy of Fallot, intrauterine growth retardation, microcephaly
|
No
|
WT1
|
11p13
|
Inactivating variants
|
Female/ Atypical
|
+/-
|
Dysgenetic
|
Late-onset renal failure Gonadoblastoma
|
Frasier
|
Inactivating variants
|
Atypical
|
+/-
|
Dysgenetic
|
Early-onset renal failure, Wilm's tumor
|
Denys-Drash
|
Inactivating variants
|
Female/ Atypical / Male with cryptorchidism
|
-
|
Dysgenetic
|
Mental retardation, Wilm's tumor, Aniridia, renal agenesis or horseshoe kidney
|
WAGR
|
WWOX
|
16q23
|
Multi-exons deletion
|
Atypical
|
+
|
Dysgenetic
|
No
|
-
|
ND: data not described
46,XY DSD ASSOCIATED WITH CHOLESTEROL SYNTHESIS DEFECTS
Smith-Lemli-Opitz Syndrome (SLOS)
This syndrome, caused by a deficiency of 7-dehydrocholesterol reductase, is the first true metabolic syndrome leading to multiple congenital malformations (179,180).
This disorder is caused by variants in the sterol delta-7-reductase (DHCR7) gene, which maps to 11q12-q13. Typical facial appearance is characterized by short nose with anteverted nostrils, blepharoptosis, microcephaly, photosensitivity, mental retardation, syndactyly of toes 2 and 3, hypotonia, and atypical genitalia. Adrenal insufficiency may be present or evolve with time. Atypical external genitalia are a frequent feature of males (71%) and ranges from hypospadias to female external genitalia despite normal 46,XY karyotype and SRY sequences. Müllerian derivative ducts can also be present (181-183). The etiology of masculinization failure in SLOS remains unclear. However, the description of patients with SLOS who present with hyponatremia, hyperkalemia, and decreased aldosterone-to-renin ratio suggest that the lack of substrate to produce adrenal and testicular steroids is the cause of adrenal insufficiency and atypical genitalia (184), although, a revision of HPA axis in these patients showed normal HPA axis function (185).
Affected children present elevations of 7-dehydrocholesterol (7DHC) in plasma or tissues. 7DHC is best assayed using Gas Chromatography/Mass Spectroscopy (GC/MS). Considering the relative high frequency of Smith-Lemli-Opitz syndrome, approximately 1 in 20,000 to 60,000 births, we suggest that at least cholesterol levels should be routinely measured in patients with 46,XY DSD. However, although frequently low, plasma cholesterol levels can be within normal limits in affected patients.
DHCR7 variant analysis can confirm a diagnosis of SLOS. The human DHCR7 gene is localized on chromosome 11q13 and contains nine exons encoding a 425 amino-acid protein (64). More than 130 different variants of DHCR7have been identified and the great majority of them are located at the exons 6 to 9 (186,187). However, the genotype-phenotype correlation in SLOS is relatively poor (188).
Currently, most SLOS patients are treated with cholesterol supplementation that can be achieved by including high cholesterol foods and/or suspensions of pharmaceutical grade cholesterol. Data suggests that early intervention may be of benefit to SLOS patients (189). Observational studies report improved growth and muscle tone and strength, increased socialization, decreased irritability and aggression in SLOS patients treated with cholesterol supplementation. However, in a group of SLOS patients’ treatment with a high cholesterol diet did not improve developmental scores (190).
Treatment with simvastatin, an HMG-CoA reductase inhibitor, aiming to block the cholesterol synthesis pathway avoiding the formation of large amounts of 7DHC/8DHC, and in this manner limiting exposure to potentially toxic metabolites in SLOS patients has been proposed. Simvastatin can also cross the blood–brain barrier and may provide a means to treat the biochemical defect present in the CNS of SLOS patients (191). A major effect of statin therapy is the transcriptional upregulation of genes controlled by the transcriptional factor SREBP, including DHCR7. Thus, if any residual activity is present in the mutant DHCR7, its upregulation could increase intracellular cholesterol synthesis. Simvastatin use in SLOS patients resulted in a paradoxical increase in serum and cerebrospinal fluid cholesterol levels (191). Randomized controlled-placebo trials were performed with simvastatin in SLOS showing significant reduction in plasmatic 7DHC associated with improvement in irritability symptoms (192). Determination of residual DHCR7 enzymatic activity may be helpful in selecting SLOS patients to be considered for a beneficial response of statins (187). Recently, promising gene therapy using an adeno-associated virus vector carrying a functional copy of the DHCR7 gene was administered by intrathecal injection in a mouse model with improvement of cholesterol levels in the central nervous system (193).
Table 4. Phenotype of 46,XY Subjects with Smith-Lemli-Optiz Sndrome
|
Inheritance
|
Autosomal recessive
|
External genitalia
|
Micropenis and/or hypospadias, hypoplastic or bifid scrotum; female
|
Müllerian duct derivatives
|
May be present
|
Wolffian duct derivatives
|
Absent to male
|
Testes
|
Scrotum, inguinal or intra-abdominal region
|
Clinical features
|
Facial and bone abnormalities. Heart and pulmonary defects. Renal agenesis. Mental retardation, Seizures, hypotonia, syndactyly of second and third toes.
|
Puberty
|
Apparently normal
|
Hormonal diagnosis
|
Low cholesterol, elevated 7-dehydrocholesterol. Decreased aldosterone-to-renin ratio
|
Gender role
|
Male
|
DHCR7 gene location
|
11q12-q13
|
Molecular defect
|
variants in DHCR7 gene
|
Treatment
|
Dietary cholesterol supplies accompanied by ursodeoxycholic acid, and statins
|
Outcome
|
Severe mental retardation
|
Dysgenetic 46,XY DSD Due to Under Expression of the DHX37 gene
46,XY gonadal dysgenesis (GD) is a heterogeneous group of disorders with a wide phenotypic spectrum, including embryonic testicular regression syndrome (ETRS) (Table 5). Screening of 87 patients with 46,XY DSD (17 familial cases from 8 unrelated families and 70 sporadic cases) using whole-exome sequencing and target gene-panel sequencing identified a new player in the complex cascade of male gonadal differentiation and maintenance - the Asp-Glu-Ala-His-box (DHX) helicase 37 (DHX37) gene (53). The variants were especially associated with ETRS (7/14 index cases; 50%). The frequency of rare, predicted-to-be-deleterious DHX37 variants in this cohort (14%) is significantly higher than that observed in the Genome Aggregation Database (0.4%; P < 0.001). Immunohistochemistry analysis in human testis showed that DHX37 is mainly expressed in germ cells at different stages of testis maturation, in Leydig cells, and rarely in Sertoli cells. Other papers confirmed these findings, associating 46,XY gonadal dysgenesis with defects in DHX37 gene (152,175).
Table 5. Phenotype of 46,XY Subjects with Gonadal Dysgenesis Due to DHX37Defects
|
Inheritance
|
Autosomal dominant
|
External genitalia
|
Micropenis, atypical genitalia or typical female
|
Müllerian duct derivatives
|
Absent uterus, Fallopian tubes may be present
|
Wolffian duct derivatives
|
Present
|
Testes
|
Abdominal region or absent
|
Histological analysis
|
Dysgenetic, no gonadal tissue
|
Puberty
|
Hypergonadotropic hypogonadism
|
Hormonal diagnosis
|
Elevated serum levels of LH and FSH; very low levels of testosterone and normal testosterone precursors levels
|
Gender role
|
Male, female, male to female
|
DHX37 gene location
|
12q24.31
|
Molecular defect
|
Heterozygous variants in DHX37 gene
|
Treatment
|
Repair of atypical genitalia; estrogen or testosterone replacement according to social sex
|
Outcome
|
Most patients keep the male social sex; some change to female social sex
|
Different modes of inheritance have been reported in familial cases of 46,XY gonadal dysgenesis, including autosomal dominant, autosomal recessive, X-linked and multifactorial inheritance (polygenic) (107,176-178). Oligogenic mode of inheritance might explain genotype/phenotype variability observed in 46,XY gonadal formation patients. Pathogenic allelic variants in NR5A1, DHX37, MAP3K1 and SRY are the most frequent molecular causes of 46,XY gonadal dysgenesis (20).
46,XY DSD DUE TO TESTOSTERONE PRODUCTION DEFECTS
Inactivating variants of human LHCG receptor (LHCGR) have been described in 46,XY individuals with a rare form of disorder of sex development, termed Leydig cell hypoplasia. These inactivating variants in the LHCGR prevent LH and hCG signal transduction and thus testosterone production both pre- and postnatally in genetic males (194).
Both hCG and LH act by stimulating a common transmembrane receptor, the LHCGR (195) LHCGR is a member of G protein-coupled receptors, which are characterized by the canonical serpentine region, composed of seven transmembrane helices interconnected by three extracellular and three intracellular loops (196,197). The large amino-terminal extracellular domain, rich in leucine-repeats, mediates the high affinity binding of pituitary LH or placental human chorionic gonadotropin (hCG) (197).
LHCGR activates the Gs protein, which determines an increase in intracellular cAMP and a subsequent stimulation of steroidogenesis in gonadal cells such as testicular Leydig cells, ovarian theca cells and differentiated granulosa cells (195,198) A secondary mechanism of LHCGR stimulation is through Gq/11 protein activation and the inositol phosphate signaling pathway (197).
The LHCGR gene is located on the short arm of chromosome 2 (2p21). It spans nearly 80 kb and has been thought to be composed of 11 exons and 10 introns. Exon 11 of the LHCGR gene encodes the entire serpentine domain as well as the carboxy-terminal portion of the hinge region (NCBI GeneID 3973; http://www.ncbi.nlm.nih.gov). The amino-terminal portion of the hinge region is encoded by exon 10 and the signal peptide and remaining portion of the extracellular domain are encoded by exons 1-9 (194,196). A novel primate-specific exon (termed exon 6A) was identified within intron 6 of the LHCGR gene. This exon is not used by the wild-type full-length receptor. It displays composite characteristics of an internal/terminal exon and possesses stop codons triggering nonsense-mediated mRNA decay in LHCGR. When exon 6A is utilized, it results in a truncated LHCGR protein (199).
In 1976, Berthezene et al. (200) described the first patient with Leydig cell hypoplasia and subsequently several cases have been reported (201-203). The clinical features are heterogeneous and result from a failure of intrauterine and pubertal virilization. A review of the literature allowed delineation of the characteristics of 46,XY DSD due to the complete form of Leydig cell hypoplasia as: 1) female external genitalia leading to female sex assignment 2) no development of sexual characteristics at puberty, 3) undescended testes slightly smaller than normal with relatively preserved seminiferous tubules and absence of mature Leydig cells, 4) presence of rudimentary epididymis and vas deferens and absence of uterus and fallopian tubes, 5) low testosterone levels despite elevated gonadotropin levels, with elevated LH levels predominant over FSH levels, 6) testicular unresponsiveness to hCG stimulation, and 7) no abnormal step up in testosterone biosynthesis precursors (194,204) (table 6).
Several different variants in the LHCGR gene were reported in patients with Leydig cell hypoplasia in both sexes (194,205).
Table 6. Phenotype of 46,XY Subjects with the Complete Form of Leydig Cell Hypoplasia
|
Inheritance
|
Autosomal recessive
|
External genitalia
|
Female, occasionally mild clitoromegaly or labial fusion
|
Müllerian derivatives
|
Absent
|
Wolffian ducts derivatives
|
Absent or vestigial
|
Testes
|
Inguinal or intra-abdominal, slightly subnormal size
|
Puberty
|
Absence of spontaneous virilization or feminization
|
Hormonal diagnosis
|
Elevated serum LH, normal or slightly elevated FSH and very low testosterone levels with normal levels of testosterone precursors
|
Gender role
|
Female
|
LHCGR gene location
|
2p21
|
Molecular defect
|
Pathogenic variants in LHCGR gene (complete inactivation) and in the internal exon 6A LHCGR(increase of nonfunctional isoform); defects in LHCGRwere not identified in several families
|
Treatment
|
Estrogen replacement at pubertal age, bilateral orchiectomy and vaginal dilation
|
Outcome
|
Female gender role and behavior, infertility
|
In contrast to the homogenous phenotype of the complete form of Leydig cell hypoplasia, the partial form features a broad spectrum, ranging from incomplete male sexual differentiation characterized by micropenis and/or hypospadias to hypergonadotropic hypogonadism without ambiguity of the male external genitalia (194,195,206,207). Testes are cryptorchidic or in the scrotum and during puberty, partial virilization occurs and testicular size is normal or only slightly reduced, while penile growth is significantly impaired. Spontaneous gynecomastia does not occur. Before puberty, the testosterone response to the hCG test is subnormal without accumulation of testosterone precursors. After puberty, LH levels are elevated as a result of insufficient negative feedback of gonadal steroid hormones on the anterior pituitary and testosterone levels are intermediate between those of children and normal males.
Several mutations in the LHCGR gene have also been identified in patients with the partial form of Leydig cell hypoplasia. Latronico et al. reported the first homozygous mutation in the LHCGR (p.Ser616Tyr) in a boy with micropenis (207). Subsequently, other milder mutations were identified in further patients with the partial form of Leydig cell hypoplasia (194,195,207). In vitro studies showed that cells transfected with LHCGR gene containing these mutations had an impaired hCG-stimulated cAMP production (195,207).
Leydig cell hypoplasia was found to be a genetic heterogenous disorder since Zenteno et al. (197) ruled out, by segregation analysis of a known polymorphism in exon 11 of the LHCG receptor gene, molecular defects in the LHCG receptor as being responsible for Leydig cell hypoplasia in three siblings with 46,XY DSD. Most inactivating mutations of the LHCGR are missense mutations that result in a single amino acid substitution in the LHCGR. In addition, mutations causing amino acid deletions, amino acid insertions, splice acceptor mutation or premature truncations of the receptor have also been reported (208). LHCGR mutations are usually located in the coding sequence, resulting in impairment of either LH/CG binding or signal transduction.
Although it is well known that hCG and LH act by stimulating a common receptor, a differential action of them in the LHCGR has been suggested. The identification of a deletion of exon 10 of the LHCGR in a patient with normal male genitalia at birth, but no pubertal development indicated that the mutant LHCGR was responsive to fetal hCG, but resistant to pituitary LH. The binding affinity of hCG for LHCGR was normal in vitro analysis, suggesting that exon 10 is necessary for LH, but not for hCG action (199).
The identification and characterization of a novel, primate-specific bona fide exon (exon 6A) within the LHCGR determined a new regulatory element within the genomic organization of this receptor and a new potential mechanism of this disorder. Kossack et al analyzing the exon 6A in 16 patients with 46,XY DSD due to Leydig cells hypoplasia without molecular diagnosis, detected mutations (p.A557C or p.G558C) in three patients. Functional studies revealed a dramatic increase in expression of the mutated internal exon 6A transcripts, resulting in the generation of predominantly nonfunctional isoforms of the LHCGR, thereby preventing its proper expression and functioning (209).
A new compound heterozygous mutation of the LHCGR, constituted by a previously described missense mutation (p.Cys13Arg) and a large deletion of the paternal chromosome 2 was identified by array-Comparative Genomic Hybridization (array-CGH) in a 46,XY infant with sexual ambiguity and low hCG-stimulated testosterone levels associated with high LH and FSH levels (200).
In addition, causative mutations in LHCGR were absent in around 50% of the patients strongly suspected to have Leydig cell hypoplasia. These findings supported the idea that other genes must be implicated in the molecular basis of this disorder.
We observed that 46,XX sisters of the patients with 46,XY DSD due to Leydig cell hypoplasia, carrying the same homozygous mutation in the LHCGR, have primary or secondary amenorrhea, spontaneous breast development, infertility, normal or enlarged cystic ovaries with elevated LH and LH/FSH ratio, normal estradiol and progesterone levels for early to mid-follicular phase, but not for luteal phase levels, confirming lack of ovulation (198,207,210). Our findings were subsequently confirmed by other authors who studied 46,XX sisters of 46,XY DSD patients with Leydig cell hypoplasia (201,202,211).
Subsequently, a novel homozygous missense mutation, p.N400S, has been identified by whole genome sequencing in two sisters with empty follicle syndrome (204).
Table 7. Phenotype of 46,XY Subjects with Partial Leydig Cells Hypoplasia
|
Inheritance
|
Autosomal recessive
|
External genitalia
|
Atypical to male
|
Müllerian derivatives
|
Absent
|
Wolffian ducts derivatives
|
Rudimentary to male
|
Testes
|
Scrotum, labial folds, or inguinal regions, normal or only slightly subnormal size
|
Puberty
|
Partial virilization without gynecomastia, discrepancy between reduced penis size and normal testicular growth
|
Hormonal diagnosis
|
Elevated serum LH levels, normal or slightly elevated FSH and low T levels with normal levels of T precursors in relation to T
|
Gender role
|
Male
|
LHCGR gene location
|
2p21
|
Molecular defect
|
Variants which confer partial inactivation of LHCGR
|
Treatment
|
Repair of the hypospadias, testosterone replacement at pubertal age
|
Outcome
|
Male gender role and behavior, possible fertility under treatment
|
46,XY DSD Due to Enzymatic Defects in Testosterone Synthesis
Six enzymatic defects that alter the normal synthesis of testosterone have been described to date (Figure 10). Three of them are associated with defects in cortisol synthesis leading to congenital adrenal hyperplasia. All of them present an autosomal recessive mode of inheritance and genetic counseling is mandatory since the chance of recurring synthesis defects among siblings is 25%.
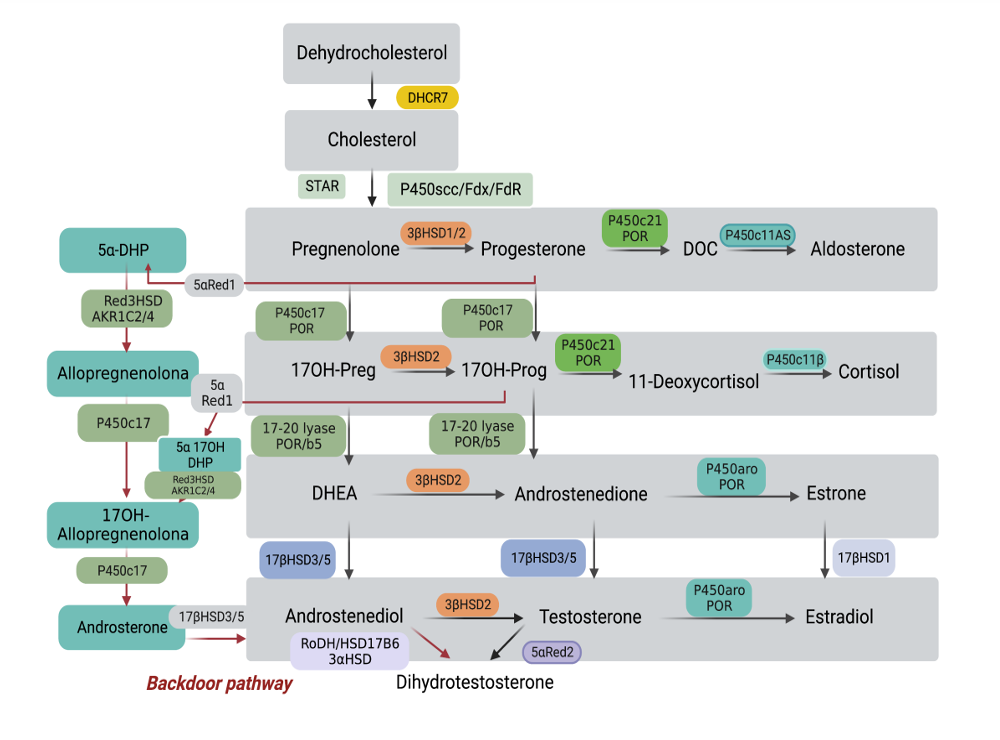
Figure 10. Standard steroidogenesis and alternative pathway to DHT synthesis.
DEFECTS IN ADRENAL AND TESTICULAR STEROIDOGENESIS
Adrenal hyperplasia syndromes are examples of hypoadrenocorticism or mixed hypo- and hyper cortico-adrenal steroid secretion. Synthesis of cortisol or both cortisol and aldosterone are impaired. When cortisol production is impaired, there is a compensatory increase in ACTH secretion. If mineralocorticoid production is impeded, there is a compensatory increase in renin-angiotensin production. These compensatory mechanisms may return cortisol or aldosterone production to normal or near normal levels, but at the expense of excessive production of precursors that can cause undesirable hormonal effects.
Lipoid Congenital Adrenal Hyperplasia due to Deficiency of the Steroidogenic Acute Regulatory Protein (StAR)
StAR is a mitochondrial phosphoprotein which facilitates the influx of cholesterol from the outer to the inner mitochondrial membrane for the subsequent action of the P450scc enzyme (212).
StAR is encoded by the STAR gene and its deficiency leads to congenital lipoid adrenal hyperplasia (CLAH), the most severe form of congenital adrenal hyperplasia (213) . Lipoid adrenal hyperplasia is rare in Europe and America, but it is thought to be the second most common form of adrenal hyperplasia in Japan where 1 in 300 individuals carries the p.Q258X variant (214).
Affected subjects are phenotypic females irrespective of gonadal sex or sometimes have slightly virilized external genitalia with or without cryptorchidism, underdeveloped internal male organs and an enlarged adrenal cortex, engorged with cholesterol and cholesterol esters (215). Adrenal steroidogenesis deficiency leads to salt wasting, hyponatremia, hyperkalemia, hypovolemia, acidosis, and death in infancy, although patients can survive to adulthood with appropriate mineralocorticoid- and glucocorticoid-replacement therapy (215).
Hormonal diagnosis is based on high ACTH and renin levels and the presence of low levels of all glucocorticoids, mineralocorticoids, and androgens.
The disease was firstly attributed to P450scc deficiency, but most of the cases studied through molecular analysis showed an intact P45011A gene and its RNA (216). Since StAR is also required for the conversion of cholesterol to pregnenolone, molecular studies were performed in StAR gene and variants were found in most of the affected patients (217) Congenital lipoid adrenal hyperplasia (LCAH) in most Palestinian cases is caused by a founder c.201_202delCT variant causing premature termination of the StAR protein (217) Histopathological findings of excised XY gonads included accumulation of fat in Leydig cells since 1 yr. of age, positive placental alkaline phosphatase and octamer binding transcription factor (OCT4) staining indicating a neoplastic potential (217).
A two-hit model has been proposed by Bose et al. (216) as the pathophysiological explanation for LCAH. In response to a stimulus (e.g., ACTH), the normal steroidogenic cell recruits cholesterol from endogenous synthesis, stored lipid droplets or low-density lipoprotein-receptor mediated endocytosis.
Subsequently StAR promotes the cholesterol transport from the outer to the inner mitochondrial membrane in which cholesterol is further processed to pregnenolone. In cells with mutant StAR (first hit), there is no rapid steroid synthesis, but still some StAR-independent cholesterol flows into the mitochondria, resulting in a low level of steroidogenesis. Due to increased steroidogenic stimuli in response to inadequately low steroid levels, additional cholesterol accumulates. Massive cholesterol storage and resulting biochemical reactions eventually destroy all steroidogenic capacity (second hit) (217). This two-hit model has been confirmed by clinical studies (218) as well as StAR knockout mice research (219).
The human STAR gene is localized on chromosome 8p11.2 and consists of seven exons (220). It is translated as a 285-amino acid protein including a mitochondrial target sequence (N terminal 62 amino acids), which guides StAR to the outer mitochondrial membrane and a cholesterol binding site, which is located at the C-terminal region. In vitro studies revealed that StAR protein lacking the N terminal targeting sequence (N-62 StAR) can still stimulate steroidogenesis in transfected COS-1 cells, whereas variants in the C-terminal region led to severely diminished or absent function (221-223). Most of the STAR gene variants associated with LCAH are located in the C-terminal coding region between exons 5 and 7 StAR related lipid transfer (START) domain (224). Mild phenotype of lipoid CAH is a recognized disorder caused by StAR variants that retain partial activity (225). Affected males can present with adrenal insufficiency resembling autoimmune Addison disease with micropenis or normal development with hypergonadotropic hypogonadism (224,225). More than 40 StAR variants causing classic lipoid CAH have been described (217,226,227), but very few partial loss-of-function variants have been reported (224-226). Therefore, there is a broad clinical spectrum of StAR variants, however, the StAR activities in vitro correlate well with clinical phenotypes (228).
Three 46,XY patients with the homozygous p.R188C STAR variant causing primary adrenocortical insufficiency without atypical genitalia were reported (229). Patients with nonclassical lipoid CAH may present with male genitalia and preserved testicular function (230).
Table 8. Phenotype of 46,XY Subjects with StAR Deficiency
|
Inheritance
|
Autosomal recessive
|
External genitalia
|
Female
Micropenis (mild form)
|
Müllerian duct derivatives
|
Absent
|
Wolffian duct derivatives
|
Absent -> hypoplastic
|
Testes
|
Small size
|
Clinical Features
|
Early adrenal insufficiency; no pubertal development; hypergonadotropic hypogonadism
|
Hormonal diagnosis
|
Elevated ACTH and renin levels; low levels of all glucocorticoids, mineralocorticoids, and androgens
|
Gender role
|
Female
Male (mild form)
|
STAR gene location
|
8p11.2
|
Molecular defect
|
Inactivating variants in STAR
|
Treatment
|
Early gluco- and mineralocorticoid replacement; estrogen replacement at pubertal age
|
Outcome
|
Infertile, female or male gender role and behavior
|
Deficiency of P450 Side Chain Cleavage Enzyme (P450scc) Due to Variants in CYP11A1
The first step in the conversion of cholesterol to hormonal steroids is hydroxylation at carbon 20, with subsequent cleavage of the 20-22 side chain to form pregnenolone. In steroidogenic tissues, such as adrenal cortex, testis, ovary, and placenta, this is the initial and rate-limiting step in steroidogenesis. This reaction, known as cholesterol side-chain cleavage, is catalyzed by a specific cytochrome P450 called P450scc or CYP11A1 encoded by the CYP11A1 gene (231).
A number of patients with CYP11A1 variants have now been described (232-235), including late-onset non-classical forms secondary to variants that retain partial enzyme activity (236,237). Clinically, these patients are indistinguishable from those with lipoid CAH, but none of them present enlarged adrenals that characterize lipoid CAH.
Analyzing infants with adrenal failure and disorder of sexual differentiation compound heterozygous variants in CYP11A1 have been identified, recognizing that this disorder may be more frequent than originally thought. The phenotypic spectrum of P450scc deficiency ranges from severe loss-of-function variants associated with prematurity, complete under androgenization, and severe early-onset adrenal failure, to partial deficiencies found in children born at term with mild masculinization and later-onset adrenal failure (236,237).
3β-Hydroxysteroid Dehydrogenase type II Deficiency
3β-hydroxysteroid dehydrogenase 2 (3βHSD2) deficiency is a rare form of congenital adrenal hyperplasia (CAH), with fewer than 200 cases reported in the world literature.
3β-HSD converts 3β-hydroxy 5 steroids to 3-keto 4 steroids and is essential for the biosynthesis of mineralocorticoids, glucocorticoids and sex steroids Two forms of the enzyme have been described in man: the type I enzyme which is expressed in placenta and peripheral tissues such as the liver and skin, and type II that is the major form expressed in the adrenals and gonads (238). The two forms are very closely related in structure and substrate specificity, though the type I enzyme has higher substrate affinities and a 5-fold greater enzymatic activity than type II (238).
Male patients with 3β-HSD type II deficiency present with atypical external genitalia, characterized by microphallus, proximal hypospadias, bifid scrotum and a blind vaginal pouch associated or not with salt loss (239,240). Precocious pubarche and gynecomastia at pubertal stage is a common phenotype in 3β-HSD type II deficiency (241).
Serum levels of Δ-5 steroids such as pregnenolone, 17OHpregnenolone (17OHPreg), DHEA, DHEAS are elevated and basal levels of 17OHPreg and 17OHPreg/17OHP ratio are the best markers of this deficiency in both prepubertal and postpubertal stage. Δ-4 steroids are slightly increased due to the peripheral action of 3β-HSD type I enzyme but the ratio of Δ-5/Δ-4 steroids is elevated. Cortisol secretion is reduced but the response to exogenous ACTH stimulation varies from decreased (more severe deficiency) to normal. At adult age, affected males can reach normal or almost normal levels of testosterone due to the peripheral conversion of elevated Δ-5 steroids by 3β-HSD type I enzyme and also due to testicular stimulation by the high LH levels (242).
The human genome encodes two functional 3βHSD genes on chromosome 1p13.1. The HSD3B2 gene is expressed in adrenal and gonads and consists of four exons coding for a 372 amino acid protein (243). To date, around 40 variants in the HSD3B2 gene have been described. Most of them are base substitutions, and they are located especially at the N-terminal region of the protein. The amino acids A10, A82, P222 and T259 could be considered a hotspot since different variants were reported in these HSD3B2 positions.
Variants abolishing 3β-HSD type II activity lead to congenital adrenal hyperplasia (CAH) with severe salt-loss (244). Variants that reduce, but do not abolish type II activity ( > 5% of wild type 3βHSD2 activity in vitro) lead to CAH with mild or no salt-loss, which in males is associated with 46,XY DSD due to the reduction in androgen synthesis (241,242,245,246). Male subjects with 46,XY DSD due 3β-HSD type II deficiency without salt loss showed clinical features in common with the deficiencies of 17β-HSD3 and 5α-reductase 2.
Most of the patients were raised as males and kept the male social sex at puberty. In one Brazilian family, two cousins with 46,XY DSD due to 3β-HSD type II deficiency were reared as females; one of them was underwent orchiectomy in childhood and kept the female social sex; the other did not undergo orchiectomy at childhood and changed to male social sex at puberty (246).
There is little data on the outcomes of 3β-HSD type II deficiency. A mixed longitudinal and cross-sectional study from a single Algerian center reported 14 affected subjects (8 females) with pathogenic variants in HSD3B2 gene (247). Premature pubarche was observed in four patients (3F:1M). Six patients (5F:1M) entered puberty spontaneously, aged 11 (5-13) years in 5 girls and 11.5 years in one boy. Testicular adrenal rest tumors were found in three boys. Four girls reached menarche at 14.3 (11-14.5) years, with three developing adrenal masses and polycystic ovary syndrome (PCOS), with radiological evidence of ovarian adrenal rest tumor in one. The median IQ was 90 (43-105), >100 in only two patients and <70 in three of them (247).
Table 9. Phenotype of 46,XY Subjects with 3β-HSD Type II Deficiency
|
Inheritance
|
Autosomal recessive
|
External genitalia
|
Atypical (proximal hypospadias, bifid scrotum, urogenital sinus), precocious pubarche
|
Müllerian derivatives
|
Absent
|
Wolffian duct derivatives
|
Normal
|
Testes
|
Well developed; generally topic
|
Clinical features
|
Adrenal insufficiency or not in infancy; virilization at puberty with or without gynecomastia
|
Hormonal diagnosis
|
Elevated basal and ACTH-stimulated 17OHPreg and 17OHPreg/17OHP ratio
|
Gender role
|
Male; female to male
|
HSD3B2 gene location
|
1p13.1
|
Molecular defect
|
Inactivating variants in HSD3B2
|
Treatment
|
Glucocorticoid replacement along with mineralocorticoids in salt-losing form; at puberty variable necessity for testosterone replacement
|
Outcome
|
Variable spermatogenesis; fertility possible by in vitrofertilization
|
Combined 17-Hydroxylase and C-17-20 lyase deficiency
CYP17 is a steroidogenic enzyme that has dual functions: hydroxylation and lyase. It is located in the fasciculata and reticularis zone of the adrenal cortex and gonadal tissues. The first activity results in hydroxylation of pregnenolone and progesterone at the C(17) position to generate 17α-hydroxypregnenolone and 17α-hydroxyprogesterone, while the second enzyme activity cleaves the C(17)-C(20) bond of 17α-hydroxypregnenolone and 17α-hydroxyprogesterone to form dehydroepiandrosterone and androstenedione, respectively. The modulation of these two activities occurs through cytochrome b5, necessary for lyase activity (248).
Deficiency of adrenal 17-hydroxylation activity was first demonstrated by Biglieri et al. (249). The phenotype of 17-hydroxylase deficiency in most of the male patients described is a female-like or slightly virilized external genitalia with blind vaginal pouch, cryptorchidism and high blood pressure, usually associated with hypokalemia. New in 1970, reported the first affected patient with atypical genitalia which was assigned to the male sex (250). The 17-hydroxylase deficiency is the second most common cause of CAH in Brazil (251).
At puberty, patients usually present sparse axillary and pubic hair. Male internal genitalia are hypoplastic and gynecomastia can appear at puberty. Most of the male patients were reared as females and sought treatment due to primary amenorrhea or lack of breast development. Genetic female patients may also be affected and present normal development of internal and external genitalia at birth and hypergonadotropic hypogonadism and amenorrhea at post pubertal age; enlarged ovaries at adult age and infarction from twisting can occur (252,253). These patients do not present signs of glucocorticoid insufficiency, due to the elevated levels of corticosterone, which has a glucocorticoid effect. The phenotype is similar to 46,XX or 46,XY complete gonadal dysgenesis and the presence of systemic hypertension and absence of pubic hair in post pubertal patients suggests the diagnosis of 17-hydroxylase deficiency (254).
Serum levels of progesterone, corticosterone, and 18-OH-corticosterone are elevated, while aldosterone, 17-OH-progesterone, cortisol, androgens and estrogens are decreased. Martin et al, performed a clinical, hormonal, and molecular study of 11 patients from 6 Brazilian families with the combined 17-alpha-hydroxylase/17,20-lyase deficiency phenotype (255). All patients had elevated basal serum levels of progesterone and suppressed plasma renin activity. The authors concluded that basal progesterone measurement is a useful marker of P450c17 deficiency and suggest that its use should reduce the misdiagnosis of this deficiency in patients presenting with male DSD, primary or secondary amenorrhea, and mineralocorticoid excess syndrome.
Excessive production of deoxycorticosterone and corticosterone results in systemic hypertension, suppression of renin levels and inhibition of aldosterone synthesis. The CYP17A1 gene, which encodes the enzymes 17-hydroxylase and 17-20 lyase, is a member of a gene family within the P450 supergene family and is mapped at 10q24.3 (254) (256). Several variants in the CYP17A1 gene have been identified in patients with both 17-hydroxylase and 17,20 lyase deficiencies (252,253,257). Four homozygous variants, p.A302P, p.K327del, p.E331del and p.R416H, were identified by direct sequencing of the CYP17A1 gene. Both P450c17 activities were abolished in all the mutant proteins but the mutant proteins were normally expressed, suggesting that the loss of enzymatic activity is not due to defects of synthesis, stability, or localization of P450c17 proteins (257).
Glucocorticoid replacement for hypertension management, gonadectomy and estrogen replacement at puberty for patients reared in the female social sex are indicated. In male patients, androgen replacement is usually necessary since they present very low levels of testosterone. These patients are very sensitive to glucocorticoids and low doses of dexamethasone (0.125-0.5 mg at night) are sufficient to control blood pressure. In some patients, however, estrogens might aggravate hypertension. The control of blood pressure can be initially achieved by salt restriction although mineralocorticoid antagonists might be necessary (257).
Table 10. Phenotype of 46,XY Subjects with 17a-Hydroxylase and 17,20-Lyase Deficiency
|
Inheritance
|
Autosomal recessive
|
External genitalia
|
Female like --> atypical
|
Müllerian duct derivatives
|
Absent
|
Wolffian duct derivatives
|
Hypoplastic --> normal
|
Testes
|
Intra-abdominal or inguinal
|
Clinical features
|
Low renin hypertension; absent or slight virilization at puberty; gynecomastia
|
Hormonal diagnosis
|
Elevated progesterone, DOC, corticosterone; low plasma renin activity low cortisol not stimulated by ACTH
|
Gender role
|
Female in most patients
|
CYP17 gene location
|
10q24.3
|
Molecular defect
|
Variants in CYP17A1 gene
|
Treatment
|
Repair of sexual ambiguity; glucocorticoid and estrogen or testosterone replacement according to social sex
|
Outcome
|
Female behavior, infertility
|
Cytochrome P450 Reductase (POR) Deficiency (Electron Transfer Disruption)
The apparent combined P450C17 and P450C21 deficiency is a rare variant of congenital adrenal hyperplasia, first reported by Peterson et al in 1985 (258). Affected girls and boys are born with atypical genitalia, indicating intrauterine androgen excess in females and androgen deficiency in males. Boys and girls can also present with skeletal malformations, which in some cases resemble a pattern seen in patients with Antley-Bixler syndrome. Findings of biochemical investigations of urinary steroid excretion in affected patients have shown accumulation of steroid metabolites, indicating impaired C17 and C21 hydroxylation, suggesting concurrent partial deficiencies of the 2 steroidogenic enzymes, P450C17 and P450C21. However, sequencing of the genes encoding these enzymes showed no variants, suggesting a defect in a cofactor that interacts with both enzymes. POR is a flavoprotein that donates electrons to all microsomal P450 enzymes, including the steroidogenic enzymes P450c17, P450c21 and P450aro (259). Shephard et al. (1989) isolated and sequenced cDNA clones that encode the rat and human NADPH-dependent cytochrome P-450 reductase and located the human gene at 7q11.2 (260).
The underlying molecular basis of congenital adrenal hyperplasia with apparent combined P450C17 and P450C21 deficiency was defined in 3 patients, who were compound heterozygotes for variants in POR (259,260). Antley-Bixler syndrome is characterized by craniosynostosis, severe midface hypoplasia, proptosis, choanal atresia/stenosis, frontal bossing, dysplastic ears, depressed nasal bridge, radio-humeral synostosis, long bone fractures, femoral bowing, phalangeal malformation (arachno-/campto-/clinodactyly, brachy-tele-phalanges, rocker bottom feet) and urogenital abnormalities (259). The occurrence of genital abnormalities in patients with Antley-Bixler syndrome, especially females was reported in 2000 (261). In a recent large survey of patients with Antley-Bixler syndrome, it was demonstrated that individuals with an Antley-Bixler-like phenotype and normal steroidogenesis have FGFR2 variants, whereas those with atypical genitalia and altered steroidogenesis have POR deficiency (262). The skeletal malformations observed in many, but not all patients with POR deficiency, are thought to be due to disruption of enzymes involved in sterol synthesis, 14α-lanosterol demethylase (CYP51A1) and squalene epoxidase, and disruption of retinoic acid metabolism catalyzed by CYP26 isoenzymes that depend on electron transfer from POR (263).
Pubertal presentations in females with congenital POR deficiency were described. Incomplete pubertal development and large ovarian cysts prone to spontaneous rupture were the predominant findings in females (264).The ovarian cysts may be driven not only by high gonadotropins but possibly also by impaired CYP51A1-mediated production of meiosis-activating sterols due to mutant POR. In the two boys evaluated, pubertal development was more mildly affected, with some spontaneous progression. These findings may suggest that testicular steroidogenesis may be less dependent on POR than adrenal and ovarian steroidogenesis (265).
Table 11. Phenotype of 46,XY Patients with POR Deficiency
|
Inheritance
|
Autosomal recessive
|
External genitalia
|
Atypical
|
Müllerian duct derivatives
|
Normally developed
|
Wolffian duct derivatives
|
Normally developed
|
Testes
|
Well developed, frequent cryptorchidism
|
Hormonal diagnosis
|
Low T and cortisol and elevated basal ACTH, Prog and 17OHP
|
POR gene location
|
7q11.2
|
Molecular defect
|
Inactivating variants of POR gene
|
Puberty
|
Spontaneous pubertal development in males
|
Gender role
|
Male
|
Treatment
|
Repair of sexual ambiguity; glucocorticoid replacement and estrogen or testosterone replacement according to social sex
|
Outcome
|
Puberty development, fertility?
|
DEFECTS IN TESTICULAR STEROIDOGENESIS
Three defects in testosterone synthesis that are not associated with adrenal insufficiency have been described: CYP17A1 deficiency, cytochrome B5 deficiency and 17-β-HSD3 deficiency
CYP17A1 Deficiency
Human male sexual differentiation requires production of fetal testicular testosterone, whose biosynthesis requires steroid 17,20-lyase activity. The existence of true isolated 17,20-lyase deficiency has been questioned because 17-α-hydroxylase and 17,20-lyase activities are catalyzed by a single enzyme and because combined deficiencies of both activities were found in functional studies of the variant found in a patient thought to have had isolated 17,20-lyase deficiency (266,267). Later, clear molecular evidence of the existence of isolated 17,20 desmolase deficiency was demonstrated (268).
The patients present atypical genitalia with micropenis, proximal hypospadias and cryptorchidism. Gynecomastia Tanner stage V can occur at puberty (268).
Elevated serum levels of 17-OHP and 17-OHPreg, with low levels of androstenedione, dehydroepiandrosterone and testosterone, are described. The hCG stimulation test results in a slight stimulation in androstenedione and testosterone secretion with an accumulation of 17-OHP and 17-OHPreg.
The CYP17A1 gene of two Brazilian 46,XY DSD patients with clinical and hormonal findings indicative of isolated 17,20-lyase deficiency, since they produce cortisol normally, were studied. Both were homozygous for missense variants in CYP17A1 (268). When expressed in COS-1 cells, the mutants retained 17α-hydroxylase activity and had minimal 17,20-lyase activity. Both variants alter the electrostatic charge distribution in the redox-partner binding site, so that the electron transfer for the 17,20-lyase reaction is selectively lost (268).
Table 12. Phenotype of 46,XY Subjects with 17,20 Lyase Deficiency
|
Inheritance
|
Autosomal recessive
|
External genitalia
|
Atypical (proximal hypospadias, bifid scrotum, urogenital sinus)
|
Müllerian derivatives
|
Absent
|
Wolffian ducts derivatives
|
Hypoplastic --> normal
|
Testes
|
In the inguinal region, small size
|
Clinical features
|
Gynecomastia variable; poor virilization at puberty
|
Hormonal diagnosis
|
Elevated 17OHP and 17OHP/A ratio after hCG stimulation and decreased A and T levels;
|
Gender role
|
Male or female
|
CYP17 gene location
|
10q24.3
|
Molecular defect
|
Variants in the redox partner binding site of CYP17A1 enzyme
|
Treatment
|
Repair of hypospadias and gynecomastia; testosterone replacement at pubertal age
|
Outcome
|
Male or female behavior
|
Cytochrome B5 deficiency (Allosteric Factor for P450c17 and POR Interaction)
In 1986, Hegesh et al described a 46,XY DSD patient with type IV hereditary methemoglobinemia (269). The patient had a 16-bp deletion in the cytochrome b5 mRNA leading to a new in-frame termination codon and a truncated protein. The etiology of 46,XY DSD in this patient was attributed to the cytochrome b5 defect since cytochrome b5 acts as an allosteric factor, promoting the interaction of. P450c17 and POR favoring 17,20 lyase reactions (270).
Two homozygous variants in CYB5 in 46,XY DSD patients with elevated methemoglobin levels but without clinical phenotype of methemoglobinemia were reported (269).
46,XY DSD due to 17β-HSD 3 Deficiency
This disorder consists of a defect in the last phase of steroidogenesis when androstenedione is converted to testosterone and estrone to estradiol. This disorder was described by Saez and his colleagues (271) and is the most common disorder of androgen synthesis, reported from several parts of the world (272,273).
There are 5 steroid 17β-HSD enzymes that catalyze this reaction (274) and 46,XY DSD results from variants in the gene encoding the 17β-HSD3 isoenzyme (275). Patients present female-like or atypical genitalia at birth, with the presence of a blind vaginal pouch, intra-abdominal or inguinal testes and epididymis, vasa deferentia, seminal vesicles and ejaculatory ducts. Most affected males are raised as females (276,277), but some have less severe defects in virilization and are raised as males (274). Virilization in subjects with 17β-HSD3 deficiency occurs at the time of expected puberty. This late virilization is usually a consequence of the presence of testosterone in the circulation because of the conversion of androstenedione to testosterone by some other 17β-HSD isoenzyme (presumably 17β-HSD 5) in extra-gonadal tissue and, occasionally, of the secretion of testosterone by the testes when levels of LH are elevated in subjects with some residual 17β-HSD3 function (274,277). However, the discrepancy between the failure of intrauterine masculinization and the virilization that occurs at the time of expected puberty is poorly understood. A limited capacity to convert androstenedione into testosterone in the fetal extragonadal tissues may explain the impairment of virilization of the external genitalia in the newborn. Bilateral orchiectomy resulted in a clear reduction of androstenedione levels indicating that the main origin of this androgen is the testis (278). 46,XY DSD phenotype is sufficiently variable in 17β-HSD3 deficiency to cause problems in accurate diagnosis, particularly in distinguishing it from partial androgen insensitivity syndrome (276,279).
Laboratory diagnosis is based on elevated serum levels of androstenedione and estrone and low levels of testosterone and estradiol resulting in elevated androstenedione/testosterone and estrone/ estradiol ratios or low (or low testosterone/androstenedione and estradiol/estrone ratios) indicating impairment in the conversion of 17-keto into 17-hydroxysteroids. Testosterone/Androstenedione ratio of 0.4±0.2 was found in prepubertal patients with 17β-HSD3 deficiency after hCG stimulation. Based on these data, a T/A ratio below <0.8 is suggestive of 17β-HSD3 deficiency (272). At the time of expected puberty, serum LH and testosterone levels rise in all affected males and testosterone levels may reach the normal adult male range (277,278).
Pitfalls in the hormonal diagnosis of 17β-HSD3 deficiency had been reported in the literature. Two of the fourteen cases of 17β-HSD3 deficiency reported from the UK database had a T/A ratio > 0.8 (276). Both patients were from a consanguineous pedigree, with two affected sisters (both assigned in the female gender) and one nephew. The former patient had atypical genitalia with proximal hypospadias and was assigned as male. The hCG test was performed at 2 years and 2 months of age, respectively, resulting in a T/A ratio of 3.4 and 1.5. Two other patients with atypical genitalia, who were also assigned in the female social sex, were evaluated at 5 months and 9.2 year of age, respectively (280). After the hCG stimulation test, there was a clear elevation of serum testosterone (measured by HPLC tandem mass spectrometry) with a small increase of the androstenedione levels resulting in a high T/A ratio (2.47 and 2.27 respectively). Sequencing of the HSD17B3 gene identified deleterious molecular defects in both alleles in both patients. The possible explanation for the normal T/A ratio in these 4 children is the individual and temporal variability in the HSD17B isoenzymes activity (280).
The disorder is due to homozygous or compound heterozygous variants in the HSD17B3 gene which encodes the 17β-HSD3 isoenzyme. Up to now, almost 40 variants in the HSD17B3 gene have been reported. These include missense, nonsense, exonic deletion, duplication and intronic splice site variants (274,277). Although allelic variants have been described throughout HSD17B3, a variant cluster region was identified in the exon 9. The 17β-HSD3 activity was completely eliminated in the majority of the HSD17B3 variants (276). Outside exon 9, the most frequent site of variant in HSD17B3 gene is the R80 in exon 3, which primarily disrupts the binding of the NADPH cofactor to the protein. The p.R80Q variant has been found in Palestinian, Brazilian, and Turkish families (281).
Most patients are raised as girls during childhood. Change to male gender role behavior at puberty has been frequently described in individuals with this disorder who were reared as females (282-285), including members of a large consanguineous family in the Gaza strip (286). In a review of all adult patients with 46,XY DSD due to 17β-HSD3 deficiency reared as female and not castrated during childhood reported until now, we found that 30 of them (61%) kept the female gender and 19 of them (39%) changed to male gender (277).
After a histological analysis of testicular tissue stained with hematoxylin-eosin from 40 reported cases of 46,XY patients with 17β-HSD3 deficiency, the prevalence of germ cell tumor was 5%, which is lower than the estimated GCT risk for some 46,XY DSD etiologies (287-289). However, the maintenance of the testes in male patients is safe if the testes can be positioned into the scrotum (277,290).
Table 13. Phenotype of 46,XY Patients with 17β-HSD 3 Deficiency
|
Inheritance
|
Autosomal recessive
|
External genitalia
|
Atypical, frequently female-like at birth
|
Müllerian duct derivatives
|
Absent
|
Wolffian duct derivatives
|
Normally developed
|
Testes
|
Well developed, frequent cryptorchidism
|
Hormonal diagnosis
|
Low T and elevated basal and hCG-stimulated A and A/T ratio
|
HSD17B3 gene location
|
9q22
|
Molecular defect
|
Inactivating variants of HSD17B3
|
Puberty
|
Virilization at puberty; variable gynecomastia
|
Gender role
|
Most patients keep the female social sex; some change to male social sex
|
Treatment
|
Repair of sexual ambiguity; estrogen or testosterone replacement according to social sex
|
Outcome
|
Male or female gender identity; in males’ fertility possible by in vitro fertilization
|
ALTERNATIVE PATHWAY TO DHT SYNTHESIS
46,XY DSD Due to 3α-Hydroxysteroid Dehydrogenase Deficiency (AKR1C2 and AKR1C4 Defects)
Back in 1972, the molecular analysis of 46,XY DSD due to isolated 17,20-lyase deficiency patients failed to find variants in the CYP17A1 (248). However, the hormonal data was inconsistent with other adrenal enzymatic deficiencies. Therefore, the alternative or backdoor pathway was considered to explain the etiology of the DSD in these patients. The backdoor pathway was firstly described in marsupials and is remarkable for having both reductive and oxidative 3α-HSD steps: the reductive reaction converts 17-OH-dihydroprogesterone (17OH-DHP) into 17OH-allopregnanolone (17OH-Allo), and the oxidative reaction converts androstanediol into DHT (291,292) (Figure 6). Therefore, synthesis of dihydrotestosterone (DHT) occurs without the intermediacy of DHEA, androstenedione or testosterone (291). All the human genes participating in the backdoor pathway have not been identified, however it has been thought that the reductive 3α-HSD activity can be catalyzed by an aldo-keto reductase called AKR1C2 (293), as well as by other enzyme, such as the oxidative 3α-HSD activity by 17β-HSD6, also called as RoDH (294)and possibly by AKR1C4 (295).
The first reported cases with isolated 17,20 lyase deficiency from 1972 (266) were found to carry variants in two aldo-keto reductases, AKR1C2 and AKR1C4 which catalyze 3α-hydroxysteroid dehydrogenase activity. The two affected 46,XY females were compound heterozygotes for AKR1C2 variants, the p.I79V/H90Q and p.I79V/N300T. However, the mutant AKR1C2 enzymes retained 22-82% of wild-type activity in vitro analysis suggesting that another gene might be involved (248). Analysis of AKR1C cDNA found that AKR1C4 was spliced incorrectly and gene sequencing displayed an intronic variant 106 bases upstream from exon 2 that caused this exon skipping. So, in this family, a digenetic inheritance was found to impair testicular synthesis of DHT during prenatal life (296).
AKR1C2 is abundantly expressed in the fetal testis, but minimally expressed in the adult testis; on the other hand, the AKR1C4 was found in fetal and adult testes at lower levels (293). Therefore, it appears that both AKR1C2 and AKR1C4 participate in the backdoor pathway to DHT in the fetal testis, and that molecular defects in these genes appear to cause incomplete male genital development (297). However, the relative roles of these two AKR1C enzymes remain unclear and testosterone levels at adult age are not available in these patients (298).
All findings described above, which substantially advanced our understanding of the underlying mechanisms of male sexual differentiation, illustrate the importance of detailed studies of rare 17,20 lyase deficiency patients.
5α-Reductase Type 2 Deficiency
A condition named pseudo-vaginal perineo-scrotal hypospadias in 46,XY individuals was reported in 1961, in which the phenotype included female-like external genitalia, bilateral testes, and male urogenital tracts with a blind-ending vagina (299). Thereafter, experimental studies showed that the male external genitalia virilization depended on the conversion of testosterone into dihydrotestosterone (DHT), an enzymatic reaction catalyzed by the 5α-reductase enzyme. Further, that enzymatic deficiency was biochemically and clinically reported in 24 individuals from the Dominican Republic and two siblings from North America (300,301). Typically, affected individuals are born with female-like external genitalia but develop clinical and psychological virilization at puberty with no gynecomastia (300). Both studies characterized this syndrome as a genetic condition with an autosomal recessive pattern of inheritance, resulting from the inability to convert testosterone into DHT. Later, two different genes encoding two 5α-reductase isoenzymes were isolated by cloning technology: the 5α-reductase type 1 and 2 (SRD5A1 and SRD5A2) (302). Allelic variants in the SRD5A2 gene were found in two individuals from Papua New Guinea with clinical features of 5α-reductase type 2 deficiency, whereas controls did not have variants in this gene, suggesting that variants in the SRD5A2 were the molecular basis of this condition (303). Further, the SRD5A2 gene was mapped at chromosome 2 (2p23), containing 5 exons and 4 introns, and encoding a 254 amino-acids protein (304).
Since then, several SRD5A2 allelic variants have been reported across the whole gene in individuals presenting this particular 46,XY DSD (305). We recently reviewed all 5α-reductase type 2 deficiency cases reported in the literature. We identified 451 cases of 5α-reductase type 2 deficiency from several countries, harboring 121 different SRD5A2 allelic variants (306). These variants have been reported in all exons of this gene, but mainly are located at exons 1 (33%) and at exon 4 (25%). Among the 254 amino acids that make up the SR5A2 protein, we found allelic variants in the SRD5A2 gene in 76 of them (306).
Regarding the SRD5A2 allelic variants, most are missense variants, but small deletions, variants at splicing sites, stop codons, small indels (n = 20) and large deletions have also been described. We also identified homozygosity in 70% of the SRD5A2 allelic variants causing 5α-reductase type 2 deficiency (306).
Neonatal diagnosis was carried out in 29.7%, whereas the remaining had the 5α-reductase type 2 deficiency diagnosis later in life. Most cases were assigned as female (69.4%), and an association between higher scores of external genitalia virilization (less virilization) and female sex assignment was identified. However, when we divided the cases into those who were diagnosed after and before 1999, the percentage of male sex assignment rose from 26.8% to 42.8%, suggesting a temporal trend pointing toward an increased likelihood of 5α-reductase type 2 deficiency patients being raised as boys (305).
Intriguingly, 5α-reductase type 2 deficiency is a condition with no genotype-phenotype correlation (307-309). This observation is based on several 5α-reductase type 2 deficiency families carrying the same genotype but presenting a broad range of external genitalia virilization. However, some SRD5A2 variants are consistent in the way they affect phenotype. It is the case of the p.Arg246Gln variant, which is associated with more external genitalia virilization (304,310-312), and also the case of both p.Gly183Ser and p.Gln126Arg variants, that are consistently reported with more severe external genitalia under-virilization (304,313-315).
The diagnosis is usually made at birth, infancy or at puberty. In the newborn, the features of 46,XY DSD due to 5a-reductase type 2 deficiency overlap with other forms of male DSD such as androgen insensitivity syndrome (partial form) and testosterone synthesis defects (304,316).
At puberty or in young adult men, the basal hormonal evaluation demonstrates normal male serum testosterone levels, low or low/normal dihydrotestosterone levels, and elevated or normal serum testosterone to dihydrotestosterone ratio (307). For appropriated use of this ratio, the testosterone levels should be in the post puberal range. Likewise, in prepubertal children, a hCG stimulation to increase serum testosterone levels is necessary (313). The biggest challenge is the diagnosis in newborns. This difficult largely arises from the fact that even when serum testosterone has undergone a neonatal surge, the ratio of serum testosterone to dihydrotestosterone may be normal, because expression of the 5a-reductase type 1 enzyme can occasionally be higher than expected (317,318).
Measurement of dihydrotestosterone is difficult because this steroid is present in very low concentrations and has a high rate of cross-reactions (319). To obtain an accurate dihydrotestosterone measurement, a precise assay must be utilized since serum testosterone levels are higher than dihydrotestosterone levels (about 10-fold). Consequently, the separation of testosterone from dihydrotestosterone is necessary to provide and accurate dihydrotestosterone measurement. Using such methodologies, the testosterone/dihydrotestosterone ratio for 5a-reductase type 2 deficiency hormonal diagnosis is generally over 18 in most cases (320,321). However, the testosterone/dihydrotestosterone ratio for 5a-reductase type 2 deficiency hormonal diagnosis has been debatable.
Another approach to 5a-reductase type 2 deficiency diagnosis is the measurement of urinary steroids by gas chromatography– mass spectrometry (GC–MS) to determine the ratio of 5a- to 5ß- reduced steroids in urine. This evaluation is very helpful for the diagnosis in subjects at prepubertal age and in orchiectomized adults. In one review, extremely low ratios of 5a- to 5ß-reduced steroid metabolites in urine were pathognomonic for 5a-reductase type 2 deficiency (322). Based on the challenges on the hormonal diagnosis, genetic analysis of the SRD5A2 gene is recommended to confirm the diagnosis [39,40].
The management in subjects with female social sex includes a careful psychological evaluation of gender identity (323). Subsequent management is similar to that in women with other forms of 46,XY DSD (324). Treatment must simulate a normal puberty pattern and low to normal estrogen doses, considering the height, and it should be administered at the age of expected puberty (10 – 12 years old). After complete breast development, adult estrogen doses are maintained continuously. Progesterone replacement is not necessary because these patients do not have a uterus (11). For women with this condition, feminizing genitoplasty is often necessary to provide an adequate vaginal opening, a functional vaginal introitus, full separation between urethral and vaginal orifice and phallic erectile tissue removal (11). Vaginal dilatation to promote vaginal length with acrylic molds is recommended when the patients decide to initiate sexual activity (325). Laparoscopic orchiectomy is recommended for all female patients to avoid virilization and gonadal malignancies. Usually, testosterone replacement is not often necessary for male patients since most retain testes and present adequate testicular function towards puberty (307). However, since the degree of virilization is usually unsatisfactory in male patients, a limited use of intramuscular testosterone or transdermal dihydrotestosterone may be helpful to improve virilization (307,319). Maximum penile length is obtained after 6 months of high dose testosterone therapy (e.g., 500 mg of testosterone cypionate per week) (319). The therapeutic penile response does not result in normal penile length in all individuals, even when initiated during childhood, and the final penile length is still below -2 SD in most patients (326). Surgical treatment consists of orthophalloplasty, scrotoplasty, resection of the vaginal pouch and proximal and distal urethroplasty. Correction of hypospadias is indicated in early childhood (up to two years old) (326).
Gender change (from female to male) is common among 5α-reductase type 2 deficiency individuals (327). It occurs in over 50% among those assigned as girls in some series (328). It may change since there is growing evidence suggesting male sex assignment for 5α-reductase type 2 deficiency newborns to avoid gender incongruence and gender dysphoria (329-331).
Regarding long-term follow up in the males from the Sao Paulo cohort, most of these subjects were satisfied with the appearance of the external genitalia and sexual life, although a small penile length made sexual intercourse difficult for some of them (326). Most of the adult males patients get married, and those reared as male report a more satisfactory quality of life than the female patients (332). Among female individuals, most describe a satisfactory sexual life, but none are married or have adopted children (333).
Table 14. Phenotype of 46,XY Subjects with 5α-Reductase 2 Deficiency
|
Inheritance
|
Autosomal recessive
|
External genitalia
|
Atypical, small phallus, proximal hypospadias, bifid scrotum, blind vaginal pouch
|
Müllerian duct derivatives
|
Absent
|
Wolffian duct derivatives
|
Normal
|
Testes
|
Normal size at inguinal, intra-abdominal region or topic
|
Puberty
|
Virilization at puberty, absence of gynecomastia
|
Hormonal diagnosis
|
Increased T/DHT ratio in basal and hCG-stimulation conditions in postpubertal patients and after hCG-stimulation in pre-pubertal subjects. Elevated 5β/5α C21 and C19 steroids in urine in all ages
|
SRD5A2 gene location
|
2 p23
|
Molecular defect
|
Inactivating variants in 5RD5A2
|
Gender role
|
Female → male in 60% of the cases
|
Treatment
|
High doses of T and/or DHT for 6 months to increase penis size
|
Outcome
|
Maximum penis size in males after treatment = 9 cm; fertility is possible by in vitro fertilization
|
46,XY DSD DUE TO DEFECTS IN ANDROGEN ACTION
Androgen Insensitivity Syndrome
Androgen insensitivity syndrome (AIS) is the most frequent etiology of 46,XY DSD individuals (334). The underlying molecular basis of AIS is variants in the androgen receptor gene (AR), which is located on the long arm of the X chromosome (Xq11-12) (335). AIS is an X-linked inherited condition, and up to 30% of AIS cases present de novovariants (334,336). Due to disruptive variants in the AR gene, affected individuals present a broad spectrum of under-virilization, which will depend upon the residual activity of the mutant AR. There are three phenotypes of AIS: complete (typically female external genitalia; CAIS), partial (a wide spectrum of external genitalia under virilization; PAIS) or mild (typically male external genitalia with further gynecomastia and/or infertility; MAIS) (337,338).
The AR contains eight exons and encodes a 920 amino-acids protein (10). The AR is composed of three major functional domains: the N-terminal transactivation domain (NTD), a central DNA-binding domain (DBD), a C-terminal ligand-binding domain (LBD), and a hinge region connecting the DBD and LBD (339,340). The main difference between the AR and other steroid receptors is the presence of a longer NTD (341). Exon 1 encodes for the NTD, while exons 2 and 3 encode for the DBD and exons 4-8 encode for the LDB (342). In the presence of androgens, the AR recruits multiple epigenetic coregulators. These co-regulators can be either co-activators or co-repressors and actupon AR influencing DNA binding, nuclear translocation, chromatin remodeling, AR stability and bridging AR with transcriptional machinery (343,344). AR coding region has two polymorphic trinucleotide repeat regions, located at exon 1, the CAG and GGC repeats (345). The length of these repeats can cause human diseases. In general, longer CAG repeats may lead to AR transactivation impairment whereas shorter CAG repeats may enhance the ARtransactivation (346). A high number of CAG repeats (>38) is the molecular cause of Spinal and Bulbar Muscular Atrophy (Kennedy’s disease) (347). This condition is characterized by severe muscular atrophy and a mild AISphenotype, including gynecomastia. On the other hand, shorter CAG repeats are related with increased risk for prostate cancer (348).
There are more than 800 variants in the AR gene reported in AIS patients (www.androgendb.mcgill.ca/; HGMD). Most of them are missense variants leading to amino acid substitutions (349). However, small indels, variants at splicing sites, premature stop codons and large deletions were also reported, most of them related to CAIS (350). Despite a well-characterized monogenic condition, AR variants are identified in 90-95% of CAIS, but only in 28-50% of PAIS (334,350). Therefore, the molecular diagnosis of PAIS individuals remains challenging. Advances in molecular biology have been helpful to clarify unusual molecular mechanisms or deep DNA alterations related to AIS. Alterations immediately upstream of the AR were identified in AIS patients without variants in the coding region of the AR, either by promoting aberrant AR transcripts, or disrupting AR expression by the insertion of a large portion of a long-interspersed element retrotransposon, which were proven to cause AIS (351,352). Rare synonymous variants within the encoding region of the AR gene were proven to play a role in AIS by disrupting splicing (353). The sequencing of intronic regions of the AR was able to identify a deep intronic variant leading to pseudo-exon activation in AIS (354). Additionally, studies involving AR variant-negative individuals with AIS revealed the deficiency of the androgen-responsive apolipoprotein D, indicating functional AIS, and epigenetic repression of the AR transcription was reported in a group of AIS variant-negative individuals, a condition defined as AIS type II (355). However, a specific role of certain coregulators in the pathophysiology of AIS is not established yet and the contribution of AR-associated coregulators in AIS remains poorly understood.
COMPLETE ANDROGEN INSENSITIVITY SYNDROME
Prenatal diagnosis of CAIS is possible and can be suspected based on the discordance between 46,XY karyotype on prenatal fetal sex determination and the identification of a female genitalia at prenatal ultrasound (356). At birth, CAIS individuals present typically female external genitalia. In childhood, the identification of an inguinal hernia in a girl may be a clinical indication of CAIS, since inguinal hernias in girls are rare (357).
At puberty, CAIS patients present with complete breast development and primary amenorrhea (358). Pubic hair and axillar hair are sparse in most of them, and Mullerian ducts are often absent in CAIS patients (337).
The endocrine evaluation after puberty shows normal or elevated serum testosterone levels and slightly elevated LH levels, whereas FSH levels can be slightly elevated, with normal presence of testosterone precursors (334).
Patients with CAIS are assigned and raised as girls and usually present a female gender identity (328,331). Estrogen replacement is recommended to induce puberty if bilateral gonadectomy has been performed before puberty. There is gonadal tumor risk in CAIS patients, but this risk is very low before puberty (359). Therefore, gonadectomy can be postponed because after puberty is complete in CAIS patients (360,361) . An increasing number of adult women with CAIS prefer to decline or delay gonadectomy for several reasons, such as fear of surgery, to avoid estrogen replacement, and expectations for future fertility (362).
Table 15. Phenotype of 46,XY Subjects with Complete Androgen Insensitivity Syndrome
|
Inheritance
|
X-linked recessive
|
External genitalia
|
Female
|
Müllerian duct derivatives
|
Absent
|
Wolffian duct derivatives
|
Absent or vestigial
|
Testes
|
Inguinal or intra-abdominal, slightly subnormal size
|
Puberty
|
Complete breast development
|
Hormonal diagnosis
|
High or normal serum LH and T levels, normal or slightly elevated FSH levels
|
Gender role
|
Female
|
AR gene location
|
Xq11-12
|
Molecular defect
|
Pathogenic allelic variants in AR gene
|
Treatment
|
Psychological support
Estrogen replacement after gonadectomy. Vaginal dilation for sexual intercourse
|
Outcome
|
Female identity, infertility
|
PARTIAL ANDROGEN INSENSITIVITY SYNDROME
Patients with PAIS have a broad spectrum of virilization impairment (337). The external genitalia ranged from predominantly female with clitoromegaly and labial fusion to predominantly male with micropenis and hypospadias. Testes are in the inguinal canal or labioscrotal folds or, less frequently, intraabdominal. At puberty, under-virilization and gynecomastia are observed (334). The final height of PAIS individuals is intermediate between the average height for control males and females. In addition, PAIS individuals presented decreased bone mineral density in the lumbar spine compared to controls (363). In male PAIS, gynecomastia is common at puberty which is helpful in the differential diagnosis from other 46,XY DSD etiologies (364).
In the endocrine analysis, serum LH levels are in the normal upper range or slightly elevated, and testosterone levels are normal or slightly elevated (334). A definitive diagnosis of PAIS is established by identifying a variant in the ARgene, but AR variants are found only in about 40% of PAIS (350).
The sex of rearing is female in half of the cases, and gender change is uncommon in PAIS patients either raised as female or male (328).
Estrogen replacement is necessary for female patients to induce adequate puberty since most female PAIS patients undergo gonadectomy in childhood (334,365). For male patients, androgen replacement, either to induce puberty or to enhance virilization post-puberty, is commonly required (11). High doses of intramuscular testosterone preparations or topical DHT can be tried for six months to improve virilization, but it is unnecessary after that (11).
If the testes are at the scrotum, gonadectomy is unnecessary in male PAIS individuals. However, bilateral gonadectomy is still recommended for female PAIS due to avoid partial virilization and due to the gonadal malignancy risk (288,337).
Table 16. Phenotype of 46,XY Subjects with Partial Androgen Insensitivity Syndrome
|
Inheritance
|
X-linked recessive
|
External genitalia
|
Broad spectrum from female with mild clitoromegaly to male with micropenis and/or hypospadias
|
Müllerian duct derivatives
|
Absent
|
Wolffian duct derivatives
|
Broad spectrum from absent or male
|
Testes
|
Eutopic, inguinal or intra-abdominal, normal or slightly subnormal size
|
Puberty
|
Gynecomastia
|
Hormonal diagnosis
|
High or normal serum LH and T levels, normal or slightly elevated FSH levels
|
Gender role
|
Female or male
|
AR gene location
|
Xq11-12
|
Molecular defect
|
Pathogenic variants in AR gene
|
Treatment
|
Females: surgical feminization, gonadectomy, replacement with estrogens at the time of puberty, vaginal dilation (if necessary)
Males: hypospadias repair, bifid scrotum; high doses of T or DHT to increase penis size
|
Outcome
|
Infertility, female or male gender role
|
46,XY DSD DUE TO PERSISTENT MÜLLERIAN DUCT
Defect in AMH Synthesis or AMH Receptor
The development of female internal genitalia in a male individual is due to the incapacity of Sertoli cells to synthesize or secrete anti-Mullerian hormone (AMH) or to alterations in the hormone receptor. Persistent Müllerian duct syndrome (PMDS) phenotype can be produced by a variant in the gene encoding anti-Müllerian hormone or by avariant in the AMH receptor. These two forms result in the same phenotype and are referred to as type I and type II, respectively (366).
AMH is a 145,000 MW glycoprotein homodimer produced by Sertoli cells not only during the period when it is responsible for regression of the Müllerian ducts but also in late pregnancy, after birth, and even, albeit at a reduced rate, in adulthood (13,367,368).
AMH is a small gene containing 5 exons, located in chromosome19p.13.3 (367) and its protein product acts through its specific receptor type 2 (AMHR2) a serine/threonine kinase, member of the family of type II receptors for TGF-β-related proteins (369).
Affected patients present a male phenotype, usually along with bilateral cryptorchidism and inguinal hernia (368). Leydig cell function is preserved, but azoospermia is common due to the malformation of ductus deferens or agenesis of epididymis. When the hernia is surgically corrected, the presence of a uterus, fallopian tubes and the superior part of the vagina can be verified.
PMDS is a heterogeneous disorder that is inherited in a sex-limited autosomal recessive manner. variants in AMHgene or AMH receptor 2 gene in similar proportions are the cause of approximately 85% of the cases of PMDS (370,371). In the remaining cases the cause of the persistent Mullerian duct syndrome is unknown (368).
Normally, AMH levels are measurable during childhood and decrease at puberty. Patients with AMH gene defects have low AMH levels since birth whereas patients with variants in AMH receptor gene have elevated AMH levels (372).
Treatment is directed toward an attempt to assure fertility in males. Early orchiopexy, proximal salpingectomy (preserving the epididymis), and a complete hysterectomy with dissection of the vas deferens from the lateral walls of the uterus are indicated (368,373).
CONGENITAL NON-GENETIC 46,XY DSD
Maternal Intake of Endocrine Disruptors
The use of synthetic progesterone or its analogs during the gestational period has been implicated in the etiology of 46,XY DSD (374). Some hypotheses have been proposed to explain the effect of progesterone in the development of male external genitalia, such as reduction of testosterone synthesis by the fetal testes or a decrease in the conversion of testosterone to DHT due to competition with progesterone (also a substrate for 5α-reductase 2 action). The effect of estrogen use during gestation in the etiology of 46,XY DSD has not been confirmed to date (375). Recently, a study in Japanese subjects supports the hypothesis that homozygosis for the specific estrogen receptor alpha 'AGATA' haplotype may increase the susceptibility to the development of male genital abnormalities in response to estrogenic effects of environmental endocrine disruptors (376).
Environmental chemicals that display anti-androgenic activity via multiple mechanisms of action have been identified. They are pesticides, fungicides, insecticides, plasticizers and herbicides. They can work as androgen receptor antagonists like pesticides, or they can decrease mRNA expression of key steroidogenic enzymes and also the peptide hormone insl3 from the fetal Leydig cells, like plasticizers and fungicides (377).
Daily exposure to residues of a fungicide (vinclozolin), either alone or in association with a phytoestrogen genistein (present in soy products), induce hypospadias in 41% of mice, supporting the idea that exposure to environmental endocrine disruptors during gestation could contribute to the development of hypospadias (378).
Supporting the idea that exposure to a mixture of chemicals can produce greater incidences of genital malformations, Rider et al examined the effects of exposure to a mixture of two chemicals that act as androgen receptor antagonists. They observed that the exposure to vinclozolin (fungicide) alone resulted in a 10% incidence of hypospadias and no vaginal pouch development in male rats, whereas procymidone, another fungicide exposure, failed to generate malformations. However, the combined exposure resulted in a 96% incidence of hypospadias and 54% incidence of vaginal pouch in treated animals. Similar results were observed in phthalate (plasticizer) mixture studies (377).
Given that severe alterations of sexual differentiation can be produced in animal laboratory studies, the question arises of what would be expected in exposed humans given that humans are exposed to mixtures of compounds in their environment.
Congenital Non-Genetic 46,XY DSD Associated With Impaired Prenatal Growth
Despite the multiple genetic causes of 46,XY DSD, around 30-40% of cases remain without diagnosis. Currently, there is a frequent, non-genetic variant of 46,XY DSD characterized by reduced prenatal growth and lack of evidence for any associated malformation or endocrinopathy (379,380). Using the model of monozygotic twins, hypospadias has now been linked to low birth weight (379). We have identified a pair of 46,XY monozygotic twins (identical for 13 informative DNA loci) born at term after an uneventful pregnancy sustained by one placenta who were discordant for genital development (perineal hypospadias versus normal male genitalia) and postnatal growth (low birth weight versus normal birth weight). No evidence for uniparental disomy was found (381). The most plausible cause of incomplete male differentiation associated with early-onset growth failure is a post-zygotic, micro-environmental factor since different DNA methylation patterns associated with silencing of genes important for sex differentiation has been shown (382).
Additionally, three cohorts of undetermined 46,XY DSD report around 30% of cases as associated with low birth weight, indicating that adverse events in early pregnancy are frequent causes of congenital non-genetic 46,XY DSD (383-385).
A genetic defect that clarifies the etiology of hypospadias was not found in 41 non-syndromic SGA children, supporting the hypothesis that multifactorial causes, new genes, and/or unidentified epigenetic defects may have an influence in this condition (385).
46,XY OVOTESTICULAR DSD
There are rare descriptions of 46,XY DSD patients with well characterized ovarian tissue with primordial follicles and testicular tissue, a condition that is histologically characterized 46,XY ovo-testicular DSD (386). The differential diagnosis of 46,XY ovo-testicular DSD with partial 46,XY gonadal dysgenesis should be performed considering that in the latter condition there are descriptions of dysgenetic testes with disorganized seminiferous tubules and ovarian stroma with occasional primordial follicles in the first years of life (46). To our knowledge there are no descriptions of an adult patient with 46,XY ovo-testicular DSD with functioning ovarian tissue, as occurs in all 46,XX ovo-testicular DSD. Therefore, the diagnosis of 46,XY ovo-testicular DSD is debatable.
Hypospadias
Hypospadias is one of the most frequent genital malformations in the male newborn and 40% of the cases are associated with other defects of the urogenital system (387). Hypospadias results from an abnormal penile and urethral development that appears to be a consequence of various mechanisms including genetic and environmental factors. It is usually a sporadic phenomenon, but familial cases can be observed, with several affected members (388,389).
The presence of hypospadias indicates an intra uterus interference in the correct genetic program of the complex tissue interactions and hormonal action through enzymatic activities or transduction signals. MAMLD1 (mastermind-like domain containing 1) has been reported to be a causative gene for hypospadias (390). It appears to play a supportive role in testosterone production around the critical period for sex development. To date, microdeletions involving MAMLD1 and nonsense and frameshift variants in the gene have been found in 46, XY DSD patients, suggesting that MAMLD1 variants cause 46,XY DSD primarily because of compromised fetal testosterone production, however, its role in the molecular network involved in fetal testosterone production is not known so far (391).
The activating transcription factor 3 (ATF3) expression was identified in the developing male urethra. Apparently ATF3 variants may influence the risk of hypospadias (392).
By definition, hypospadias is a form of 46,XY DSD and although most of the patients maintain fertility and masculinization at puberty, their testicular function should be assessed to rule out causes such as defects in testosterone synthesis and action, which require hormonal treatment and genetic counseling in addition to surgical treatment.
GONADAL TUMOR DEVELOPMENT IN 46,XY DSD PATIENTS
Any disturbance in the gonadal development, including testicular descent, increases the risk of developing gonadal malignancies (288). For inherited disturbances in gonadal development or endocrine alterations, patients with 46,XY DSD are at increased risk of developing type II germ cell tumors (GCT) (289). In testicular tissues, GCTs comprise both premalignant conditions, such as germ cell neoplasia in situ (GCNIS) and malignant invasive germ cell tumors, including seminomas and non-seminomas (393).
The term GCNIS was introduced in the 2016 WHO classification of urological tumors to define precursor lesions of invasive GCTs, since GCNIS has the potential to develop into several types of GCTs (394,395). GCNIS cells are fetal gonocytes present in the seminiferous tubules arrested during gonadal development that failed to mature into spermatogonia (396). GCNIS are often detected in testicular tissues from 46,XY DSD subjects (397). It is estimated that 50% of GCNIS progress to an invasive GCT in five years (396,398,399).
A high risk of GCT is found when sex determination is disrupted at an early stage of Sertoli cell differentiation (due to abnormalities in SRY, WT1, SOX9) (289,397,400). For that reason, specific etiologies of 46,XY DSD (401) have a significant risk factor for GCT development (393). Early Sertoli cell development is also disturbed in patients with 45X/46,XY mosaicism (402). The presence of the well-defined Y chromosome region, known as the gonadoblastoma Y locus (GBY), is a prerequisite for malignant transformation. Among the genes located in the GBY region the testis-specific protein Y (TSPY) seems to be the most significant candidate gene for the tumor-promoting process (288,403). The presence of undifferentiated gonadal tissue containing germ cells that abundantly express TSPY has also been identified as a gonadal differentiation pattern bearing a high risk for GCT development (404). Prolonged expression of OCT3/4 (POU5F1) and the stem cell factor KITL after one year of age are also estimated to play a role in GCNIS/GCT development. Other factors implicated in that risk include MAP3K1 variants in 46,XY patients with gonadal dysgenesis due to MAPK signaling pathway upregulation and loss of androgen receptor function in patients with androgen insensitivity syndrome (289,405). Additionally, gonads at the abdominal region are at higher risk of GCNIS/GCT development than those appropriately positioned (393,406).
Unfortunately, GCNIS/GCT screening is challenging due to a lack of a predictive factor or a biomarker with adequate sensitivity and specificity (407). As far as imaging is concerned, ultrasound (US) is more sensitive than MRI at identifying dysgenetic gonads, but MRI showed better sensitivity and specificity than US at localizing non-palpable gonads (408). However, both imaging techniques are poor at identifying GCNIS/GCT, since MRI failed to identify GCNIS in patients with CAIS and the US only identified one out of ten malignant lesions in 46,XY DSD people (409). There are serum markers that are associated with GCT in non-DSD people, such as alpha-fetoprotein, beta-hCG, and lactate dehydrogenase, but there is poor evidence about how useful they are for GCT screening in 46,XY DSD individuals (410). An interesting perspective for GCT screening are microRNAs (miRNA), since some miRNA clusters are expressed in the presence of GCT (411). For non-DSD people, microRNAs are more sensitive than serum markers and imaging to detect GCT. Noteworthy, GCNIS also expresses some embryonic-type miRNAs (miR-371-3, miR-302, and miR-367) that are also expressed by GCTs (410,412). Therefore, they have the potential to serve as a biomarker even for GCNIS(407).
Overall, neoplastic transformation of germ cells in dysgenetic gonads (gonadoblastoma and/or an invasive germ cell tumor) occurs in 20-30% of 46,XY DSD individuals, but the risk varies among 46,XY DSD etiologies (413). Individuals with Denys-Drash syndrome (40%), Frasier syndrome (60%), and gonadal dysgenesis (15 - 35%) have the highest risk of GCNIS/GCT among 46,XY DSD etiologies (413). On the other hand, individuals with CAIS (at prepubertal age) and ovotestis DSD have lower risk of GCNIS/GCT (414). The age matters in the estimation of GCNIS/GCT risk. For example, it is as low as 1.3% in CAIS individuals before puberty, but it can reach 33% thereafter (287,415).
For 46,XY DSD subjects, gonadectomy is classically recommended to avoid GCNIS/GCT development, preventing additional therapies and related risks (290). Despite a very effective strategy to avoid GCNIS/GCT, gonadectomy leads to hypogonadism and infertility.
Regarding the time for gonadectomy, bilateral gonadectomy should be performed in early childhood in 46,XY DSD patients with gonadal dysgenesis, females with Y chromosome material, and patients with androgen biosynthesis defect, unless the gonad is functional and easily accessible to palpation and imaging studies, which should be performed yearly (11,289). Although data are limited, in the androgen insensitivity syndrome the risk seems to be markedly lower in the complete form before puberty than in the other 46,XY DSD (416). Therefore, gonadectomy can be postponed until puberty is complete in CAIS individuals (417). Unfortunately, the GCNIS/GCT risk for other causes of 46,XY DSD patients, such as Leydig Cell Hypoplasia and 5 alpha reductase type 2 deficiency has not been estimated yet.
Rarely, gonadal tumors can produce sexual steroids (418). In those cases that are able to produce estrogens, spontaneous breast development may be a clinical sign that suggests the presence of an estrogen-secreting gonadal tumor, and bilateral gonadectomy is indicated even at early childhood, regardless of the 46,XY DSD etiology.
Overall, 46,XY DSD patients are at increased risk for gonadal malignancy which seems to be related to 46,XY DSD etiology. While it is clear that prepubertal CAIS patients are at low risk for GCT and 46,XY DSD individuals harboring WT1 variants present a high risk for GCT development, the real GCT risk for other 46,XY DSD etiologies is not that clear (413). In the absence of a reliable predictive factor or biomarker of GCNIS/GCT as well as appropriate recommendations for GCT screening, bilateral gonadectomy will still be recommended for most 46,XY DSD etiologies.
FERTILITY IN PATIENTS WITH 46,XY DSD
Most 46,XY DSD individuals face infertility due to abnormal gonadal development, endocrine disturbances, anatomical issues, or prophylactic gonadectomy for malignancy risk (419). However, there has been growing evidence showing that fertility is relevant for several 46, XY DSD people, in addition to the possibility of delaying gonadectomy in some 46,XY DSD etiologies (420). In parallel, fertility preservation technologies have been improved in recent years along with a better social perception of non-traditional family structures (421,422).
In 46,XY DSD, the fertility potential varies depending on the underlying etiology as well as the severity of the condition (421). In this sense, all options for fertility should be discussed considering the 46,XY DSD etiology or the gonadal structure and internal genitalia in those in whom the 46,XY etiology is unknown (420).
For example, individuals with complete gonadal dysgenesis possess uterus, despite lacking gametes (423). Therefore, pregnancy by oocyte donation is an alternative for these patients. On the other hand, male individuals with partial and mild androgen insensitivity often present oligospermia, but biological fertility is possible (334,338).
Overall, there is limited literature about fertility potential among 46,XY DSD people. Successful biological fertility was obtained in a man with PAIS after prolonged high-dose testosterone therapy followed by intracytoplasmic sperm injection (424). The possibility of fertility seems to be more frequent among MAIS since there are six cases of successful fertility (338). There are few reported cases of successful pregnancies and live births in men with 5RD2 deficiency, both spontaneous and with assisted reproductive technology (319,425-427). Biological fertility has also been documented in individuals with nonclassical congenital lipoid adrenal hyperplasia, 3b-HSD2 deficiency, and LHCG receptor defect (420). Conversely, there are no reported cases of biological fertility in individuals with classic congenital lipoid adrenal hyperplasia, cytochrome p450 oxidoreductase deficiency, complete CYP17A1 deficiency, 17b-HSD3 deficiency, and CAIS (419,428).
To estimate fertility potential, a pilot study evaluated the presence of germ cells and the germ cell density in individuals with several 46,XY DSD etiologies (429). In six patients with CAIS, all presented Sertoli Cell nodules and hyperplasia, but germ cells were detected in areas between nodules. All six patients with mixed gonadal dysgenesis and two with ovo-testicular DSD presented germ cells, and ten out of twelve 46,XY DSD patients with unknown etiology presented germ cells in their gonads. On the other hand, germ cells were not found in any of the patients with either complete or partial gonadal dysgenesis. However, the number of germ cells was inversely correlated with age, suggesting that the gonadectomy delay may decrease fertility potential. It needs to be confirmed by more extensive studies, but it indicates that 46,XY DSD fertility potential may be greater than previously thought.
As far as desire for fertility is concerned, a large follow-up study included 1,040 DSD individuals to investigate their fertility preferences (430). The authors reported that 55% of patients expressed a desire to have had fertility treatments in the past or have it in the future, and 40% mentioned that they would like to try new fertility treatment techniques. Additionally, CAIS women reported the possibility of future fertility as one of the reasons to keep their gonads (362).
Indeed, fertility preservation has been primarily assessed in oncology to preserve patients' fertility under gonadotoxic treatments (431). In this sense, cryopreservation of postpubertal testicular tissue is helpful to keep fertility potential in patients having gonadectomy or those before gonadotoxic treatment. As an alternative, cryopreservation of immature testicular tissue containing spermatogonial cells or spermatogonial stem cells can be offered to prepubertal patients (432). These techniques could also be considered for 46,XY DSD patients.
In summary, addressing fertility is essential in 46,XY DSD management. The fertility potential must be discussed considering the 46,XY DSD etiology and the patient’s desire. As assisted fertility and preservation techniques improve, these advancements should be offered and accessible to all 46,XY individuals.
46,XY GENDER IDENTITY DISORDERS
Transgender Women are characterized by the wish to live as members of the female sex with conviction and consistently and progressively efforts to achieve such state. 46,XY gender identity disorders are more frequent among the male sex, although it also occurs in the female sex. Its first manifestations usually start during childhood. If it has a biological basis is still unknown, but some hormonal alterations during intrauterine life and familial factors before and after birth cannot be ruled out (433).
The term used to name men and women who live a relevant incongruence between their gender identity and their inborn physical phenotype has changed over time. The term “trans-sexualism” was coined by Hirschfeld in 1923 and was adopted by the International Classification of Diseases – version 10 (ICD-10). The American Psychiatric Association, in its 4th edition, adopted “gender identity disorder” to define persons who are not satisfied with their biological gender (Association, American Psychiatric. "Diagnostic and statistical manual of mental disorders (2000).
Finally, the current classification system of the American Psychiatric Association (DSM-5) replaced the term “gender identity disorder” with “gender dysphoria” and the upcoming version of International Classification of Diseases – version 11 (ICD-11) has proposed the term “gender incongruence” (434).
In this chapter we will use the current DSM-5 term, “gender dysphoria”. To refer to male to female gender-dysphoric persons we will use the term transgender woman (American Psychiatric Association. Diagnostic and Statistical Manual of Mental Disorders, Fifth Edition - DSM-5; 2013). Therefore, the term “transgender woman” refers to all 46, XY individuals with typical male phenotype who wish to live and be accepted as a female.
Higher prevalence of addictions and suicidal thoughts or suicide attempt than those observed in the general population, revealed the need for early care of these patients by health professionals. Among transgender women, total mortality was 51% higher than in the general population, mainly from increased mortality rates due to suicide, acquired immunodeficiency syndrome, cardiovascular disease, drug abuse, and unknown cause (435). Based on these data, supervised gender-affirming treatment for gender dysphoric persons is crucial because they are at increased risk of committing suicide and self-harm (436).
Management of Adult Transgender Women
As proposed by the Harry Benjamin International Gender Dysphoria Association, now known as World Professional Association for Transgender Health (WPATH), the process of gender-affirming treatment should be given by a multi and interdisciplinary team, in which the endocrinologist has a key role (437).
The interdisciplinary team should consist of a psychologist, a psychiatrist, an endocrinologist, and a surgeon, at least (438). It would be ideal that they all participate in an integrated and consistent way across all the steps of the treatment (439).
The mental health professionals (psychologist and psychiatrist) make a distinction between gender dysphoria and conditions with similar features (body dysmorphic disorder and body identity integrity disorder), decide whether the individuals fulfill ICD-11 and DSM-5 criteria, recommend the appropriate treatment and follow-up before, during and after gender-affirming treatment. The endocrinologist will inform about the possibilities and limitations of all sorts of treatment, initiate and monitor the cross-sex hormonal treatment and participate in the indication of gender-affirming surgery. At the final step, the surgeon performs surgical procedures of the treatment (439).
DIAGNOSTIC ASSESSMENT AND MENTAL HEALTH CARE
Psychological evaluation of persons with gender dysphoria should consider the evolution of the individual as whole, using psychological assessment instruments, such as: freely structured interviews and patterned psychological assessment instruments. For the structured interview, we use a specific questionnaire developed by our mental health professionals that covers childhood, adolescence, and adulthood aspects.
During the psychotherapeutic follow up, besides offering an ideal condition for elaborating conflicts and issues regarding gender identity, other variables should be considered, such as individual general state of mental health, ability and manner of conflict resolution, quality of interpersonal relationships, ability to deal with frustrations and limitations, particularly regarding to surgery’s esthetic and functional results idealization. It is recommended that the relatives and/or spouses are invited for interviews to clear them up upon the offered treatment.
HORMONAL THERAPY FOR ADULT TRANSGENDER WOMEN
Endocrinologists have the responsibility to confirm that persons fulfill criteria for hormonal treatment and clarify the consequences, risks, and benefits of treatment.
Hormone therapy must follow well-defined criteria. The person with gender dysphoria has to: 1) demonstrate knowledge and understanding of the expected and side effects of cross-sex hormone use; 2) complete a real life experience in the gender identity for at least three months, or psychotherapy for a period determined by the mental health professional to consolidate gender identity; and 3) be likely to take hormones appropriately (439).
There are two major goals of hormonal therapy: 1) to replace endogenous sex hormone levels and, thus, induce the appearance of sexual characteristics compatible with female gender identity; 2) to reduce endogenous sex hormone levels and, thereby, the secondary male sexual characteristics and 3) to establish the ideal hormones dosage which allows physiological hormone serum levels compatible with female gender identity by adopting the principles of hormone replacement treatment of hypogonadal patients (439,440).
Hormone therapy provides a strong relief from the suffering caused by the incongruence of the phenotype with the gender identity.
In our clinical practice, we observe that most transgender women consume very high doses of female sex hormones, guided by their wish to obtain fast breast development, and reduce facial hair. However, high doses of hormones are not necessary to achieve the desired effects and are frequently associated with undesirable side effects.
The chosen hormone to induce female secondary sexual characteristics are the estrogens. Several pharmaceutical estrogen preparations, including oral, injectable, transdermal, and intravaginal forms associated or not with progesterone are available. Due to the higher cost of the transdermal preparations, the oral route is the most widely used. Nevertheless, the transdermal route is recommended for transgender women over 40 years of age due to the lower association of transdermal 17β-estradiol replacement with thromboembolic events (441).
Anti-androgens are used as adjuvants to estrogen, especially in the reduction of male sexual characteristics and the suppression of testosterone to levels compatible with the female sex. Cyproterone acetate blocks testosterone binding to its receptor, and in a dose of 50-100 mg/day associated with estrogen can maintain testosterone in female levels in transgender women (442).
At the time, most of the patients in our clinic used conjugated equine estrogens at a dose of 0.625-1.25 mg/day associated with 50 mg/day of cyproterone acetate for an average period of 11 years. At clinical examination we observed satisfactory breast development, decrease of spontaneous erections, thinning of facial and body hair (especially after association with cyproterone acetate), body fat redistribution, enlargement of the areola and nipple and reduction of testicular volume (440).
Testosterone levels remained at pre- or intra-pubertal female range (< 14-99 ng/dL) in all patients; LH levels were pre-pubertal (<0.6-0.7 U/L) in 72% of the cases, and the FSH levels were suppressed (<1.0 U/L) in 40% of cases. Therefore, daily use of oral conjugated estrogens at low doses in association with cyproterone acetate is effective in suppressing the hypothalamic-pituitary-testicular axis in transgender women (440).
Venous thromboembolism may be a serious complication related to estrogen therapy, particularly during the first year of treatment, when the incidence of this event is 2-6% falling to 0.4% in the second year, significantly higher when compared to the overall young population (0.005 to 0.01%/year). This high incidence of thromboembolic events in transgender women seems to be more associated with ethinyl estradiol than natural oral or transdermal estrogens (441). All patients on estrogen therapy have a mild prolactin level increase. However, a small percentage of these subjects have galactorrhea. In our cohort, two patients had macroprolactinoma, which totally regressed with dopamine agonist treatment. Both had previously used high doses of estrogen (443). Endocrinologists should monitor weight, blood pressure, breast enlargement, body hair involution, body fat redistribution and testicular size every six months. The laboratory evaluation should include measurement of LH, FSH, testosterone, estradiol, prolactin, liver enzymes, complete blood count, coagulation factors, and lipid profile. Bone densitometry and breast ultrasound should be performed yearly.
After surgery in patients over 50 years old, the measurement of PSA should be conducted yearly (440).
The current key issues include avoiding supraphysiological doses of estrogen and the use of ethinyl estradiol. The preference should be given to conjugated estrogens or transdermal natural estrogen, especially in patients over 40 years of age (444). Hormone therapy provides a strong relief from the suffering caused by gender dysphoria. (440).
MANAGEMENT OF PATIENTS WITH 46,XY DSD
It is important to stress that the treatment of 46,XY DSD patients requires an appropriately trained multi-disciplinary team. Early diagnosis is important for better outcomes and should start with a careful examination of the newborn’s genitalia at birth (445-447).
Psychological Evaluation
It is of crucial importance to treat DSD patients (448). Every couple that has a child with atypical genitalia must be assessed and receive counseling by an experienced psychologist, specialized in gender identity, who must be act as soon as the diagnosis is suspected, and then follow the family periodically, more frequently during the periods before and after genitoplasty (449,450).
Parents must be well informed by the physician and psychologist about sexual development (451). A simple, detailed, and comprehensive explanation about what to expect regarding integration in social life, sexual activity, need of hormonal replacement and surgical treatment and fertility issues should also be discussed with the parents, before sex assignment (11).
The sex assignment must consider the etiological diagnosis, external genitalia, cultural and social aspects, sexual identity and the acceptance of the assigned gender by the parents (452). In case parents and health care providers disagree over the sex of rearing, the parents’ choice must be respected. The affected child and his/her family must be followed throughout life to ascertain the patient’s adjustment to his/her social sex.
Hormonal Therapy
Sex steroid replacement is an important component of management for some types of 46,XY DSD (453,454). The goals of replacement include induction and maintenance of secondary sex characteristics as well as other aspects of pubertal development including growth. Bone mineral optimization and promotion of uterine development may also be helped by treatment with sex steroids for some patients. Hormone replacement can also impact psychosocial and psychosexual development, as well as general wellbeing, in positive ways for some people (455,456). Induction and maintenance of pubertal development is necessary in most patients affected by 46,XY DSD regardless of male or female rearing; however, specific indications depend on the underlying etiology of the condition.
FEMALE SOCIAL SEX
The purpose of the hormonal therapy is the development of female sexual characteristics and menses in the patients with uterus. There are several options available for estrogen replacement as well as different combinations and doses of progestins (457) however, 17β-estradiol (oral or transdermal) is preferred. Estrogen therapy should be initiated at a low dose (1/6 to 1/4 of the adult dose) to avoid excessive bone maturation in short children and increase gradually at intervals of 6 months. Doses can then be adjusted to the response (Tanner stage, bone age), with the aim of completing feminization gradually over a period of 2–3 years. In 46,XY females with tall stature, adult estrogen dosage is recommended to avoid high final stature. Transdermal delivery avoids hepatic first-pass metabolism resulting in less thrombogenicity and more neutral effects on lipids (458,459). It is also easier to administer small doses of estrogen by cutting up a patch or by using a metered-dose gel dispenser. An initial recommended dose of oral 17-βestradiol is 5 μg/kg daily, titrated every 6–12 months to an additional 5 μg/kg daily until an adult dose of 1–2 mg daily is achieved (459) .
In case of transdermal replacement, the initial recommended dose for the 17-β estradiol patch is 3.1–6.2 mg/24h overnight (1/8–1/4 of 25 mg/24h patch). Transdermal doses can increase 3.1–6.2 mg/24h every 6 months until an adult dose of 50-100 mg/24 h twice a week is achieved (460) Once breast development is complete, an adult dose can be maintained continuously (11). For patients who do not have a uterus, estrogen alone is indicated (458,461). Progesterone is needed to induce endometrial cycling and menses in patients with a uterus. For the latter group, medroxyprogesterone acetate (5 to 10 mg/day) or micronized progesterone (200 mg/day from the 1st to the 12th day of each month) are appropriate to maintain uterine health.
Some females with CAIS report decreased psychological wellbeing and sexual dissatisfaction following bilateral gonadectomy and subsequent estrogen replacement (462,463).
Testosterone treatment has been proposed as an alternative to estrogen for hormone replacement in these women and such treatment improves sexual desire (464). However, long-term follow-up studies on the impact of T replacement on additional psychological measures, as well as on bone metabolism and cardiovascular outcomes, are needed (465).
The dilation of the blind vaginal pouch with acrylic molds (325) or exceptionally surgical neovagina promote development of a vagina adequate for sexual intercourse after 6-10 months of treatment when patients desire to initiate sexual activity (466).
MALE SOCIAL SEX
For those raised male, T replacement should strive to mimic masculine pubertal induction between 10 and 12 years of age, provided the child’s predicted height and growth are normal and he indicates a desire and readiness for puberty (5). Intramuscular, short-acting injections of T esters are the most suitable formulation to induce male puberty, although other options include oral T undecanoate and transdermal preparations (467,468).. The initial dose of short-acting T esters is 25–50 mg/month intramuscularly, with further increments of 50- 100 mg every 6–12 months, thereafter. After reaching a replacement dose of 100–150 mg/month, the delivery interval can decrease to every two weeks.
An adult dose of 200-250 mg every two weeks (short-acting T esters), 1000 mg every 10-14 weeks (long-acting T esters), or 50-100 mg for T gel or other transdermal preparations applied topically are effective to maintain male secondary sex characteristics (12,468). Monitoring of T levels should be performed on the day preceding the next hormone administration, and serum levels should fall just above the lower limit of the normal range for eugonadal men.
In male patients with androgen insensitivity, higher doses of testosterone esters (250-500 mg twice a week) are used to increase penile length and male secondary characteristics. Maximum penis enlargement is obtained after 6 months of high doses and after that, the normal dosage is re-instituted (272,313). The use of topical DHT gel is also useful to increase penile length with the advantage of not causing gynecomastia.
Glucocorticoid Replacement
It is necessary for 46,XY DSD patients with classical forms of congenital lipoid adrenal hyperplasia, POR, 3β-HSD type II deficiency to receive glucocorticoid replacement for adrenal insufficiency and in 17α-hydroxylase/17,20-lyase deficiency for hypertension management (469) (267).
Mineralocorticoid replacement is also required for 46,XY DSD salt-losing patients (470).
Surgical Treatment
Surgical approach for 46,XY DSD patients includes: gonadal management, removal of internal structures that disagree with the social sex and reconstruction of the atypical external genitalia. Genital reconstruction involves the feminization or masculinization of external genitalia; these procedures are being widely discussed and controversy continues over the ideal age for genital surgery (471,472). There is a lack of data concerning this issue: a survey with 459 individuals (≥ 16 years) with a DSD diagnosis concerning patients desire about timing of genital surgery was published (473). A total of 66% of individuals with CAH and 60% of those with 46,XY DSD thought that infancy or childhood were the appropriate age for genital surgery. This report concluded that case-by-case decision-making is the best approach (473). In our experience, patients submitted to surgery in adulthood, preferred surgery in infancy and none of the patients operated during childhood regretted the surgery at that age (474).
Laparoscopy is the ideal method of surgical treatment of the internal genital organs in patients with 46,XY DSD (475). In these patients, the indications for laparoscopy are the removal of gonads and ductal structures that are contrary to the assigned gender and the removal of dysgenetic gonads, which are nonfunctional and present potential for malignancy. In addition to being a minimally invasive surgery, one of the main advantages of this method is the lack of scars.
Feminizing genitoplasty includes the reduction of enlarged clitoral size, opening the urogenital sinus to separate the urethra from the vaginal introitus, and constructing labioscrotal folds. Feminizing techniques have evolved over time to achieve better cosmetic outcomes (476,477). Many techniques have been proposed to separate the urethra from the vaginal introitus and bring both to the surface of the perineum. Fortunoff and Latimer in 1964 described the most commonly used technique until the present day, using an inverted U-shaped perineal skin flap to enlarge the vaginal introitus allowing adequate menstrual flow and future sexual activity (478). Failure to interposing an adequate flap will result in persistent urogenital sinus or vaginal introitus stenosis, requiring later revision (479). Vaginal dilation with acrylic molds in patients with short vagina or introitus stenosis showed to be a good treatment choice when these patients wished to start sexual intercourse, resulting in better outcomes (325). To reduce clitoral enlargement a number of techniques were proposed during the years (480). Kogan described preserving the neuro-vascular bundle attached to the dorsal portion of the tunica albuginea to protect the nerves and blood supply (481) and this is the technique of choice. The redundant clitoral skin obtained during clitoroplasty is used to create the labia minora; this skin is divided longitudinally and then sutured along either side of the vagina. When necessary, the reduction of labioscrotal folds is performed to create the labia majora, often using a Y-V plasty technique (482).
The most common surgical complications, in feminizing genitoplasty, includes: clitoral ischemia or necrosis that can rarely occur in patients with high grade of virilization; introitus stenosis or vaginal stenosis particularly when the confluence of the vagina and urethra is far from the perineum surface and urinary infections mostly observed in patients with persistence of urogenital sinus (471,479,480).
In order to minimize surgical complications and dissatisfaction in adulthood, only skilled surgeons with specific training should perform these procedures in the DSD patients (8). In our experience, the single-stage feminizing genitoplasty consisting of clitoroplasty with the preservation of dorsal nerves and vessels and ventral mucosa, vulvoplasty and Y-V perineal flap, followed by vaginal dilation with acrylic molds, allowed good cosmetic and functional results (483).
For the males, masculinizing procedures aims to allow the patient to have micturition standing up without effort with a straight and wide stream and to have a satisfactory sexual life with straight erections. The genital surgery consists in correction of hypospadias and scrotal abnormalities, relocation of the testes to the scrotum or removal when dysgenetic, and resection of Mullerian remnants (326,484). Correction of hypospadias includes correction of phallic curvature (orthophalloplasty) and construction of a urethra to the tip of the glans (urethroplasty). Preoperative administration of testosterone is indicated for patients with a small penis (485). Usually, multistage procedures are preferred for male genital reconstruction in DSD patients, due to the severe under virilization represented by proximal hypospadias with severe curvature. The first stage repair consists in ortho-phaloplasty and scrotoplasty (486) (487). The second stage is performed 6-9 months later and consists in urethroplasty). The two-stage approach typically results in better cosmetic outcomes and fewer postoperative complications for patients with severe hypospadias and significant chordee (326,487-489). The most frequent complication in correction of hypospadias is urethral fistula (23%) followed by urethral strictures (9%) and diverticula (4%) (490). This frequency is highly variable in the literature (490). Fistula can be observed just after surgery or months later, but urethral stenosis in some cases can occur several years after surgery. Reoperations are necessary to correct fistula, diverticula and particularly to treat severe urethral strictures. The buccal mucosa graft is commonly used to enlarge the urethra in these cases (490). For patients with undescended testes, simultaneous orchidopexy may be performed. The surgical treatment of gonads of 46, XY DSD patients aims to preserve testicular function (production of testosterone and sperm) and prevent malignancy (288,360,491). Finally, gonadectomy is recommended for patients at risk for neoplastic transformation of germ cells (gonadoblastomas and/or an invasive germ cell tumor) in dysgenetic gonads (287).
When gonadectomy is recommended, patients may then choose to have a testicular prosthesis placed in the scrotum (492).
Müllerian structures are rudimentary in some patients and present as a cystic prostatic utricle. These utricles may be left in situ when asymptomatic, but in cases of recurrent urinary tract infection, stones, or significant post-void urethral dribbling due to urinary pooling, they can be removed either laparoscopically or through a sagittal posterior incision of the perineum (475). With either approach, great care must be taken to prevent injury to the vas deferens, seminal vesicles and pelvic nerves so as to avoid subsequent infertility, erectile dysfunction and urinary incontinence (488,493). Late evaluation of 46,XY DSD patients operated in childhood due to proximal hypospadias reveals that many felt that their genitals had an unusual appearance or presented some degree of urinary or sexual dysfunction (494). Objectively, most DSD patients have a penile length below the -2.0 SD (5.2 ± 2.0 cm) (326). Dysfunctional voiding and lower urinary tract symptoms are also more frequent in these patients than in controls (495). However, between 55.6 and 91% of these patients after genitoplasty were satisfied with their overall sexual function after genitoplasty, when considering sexual contacts, libido, erections, orgasm, as well as size of the penis and volume of ejaculation (326),(494),(496),(497),(498). The long-term outcomes were evaluated for a long time concerning functional and cosmetic results that could be analyzed by objective criteria. The subjective long-term evaluation analyzing psychological and sexual implications in quality of life were often neglected in the past, but is being currently explored (332) (499) (500). Jones et al reported that 81% were satisfied with their genital appearance and that 90% were satisfied with their overall body image (500). Most of our patients were satisfied with their genital appearance and present satisfactory sexual performance as long as they present a penis size of at least 6 cm (326).
ACKNOWLEDGMENT
The authors would like to thank the postgraduate students Nathalia Lisboa Gomes and Jose Antonio D Faria Junior for their help in the update of this chapter.
REFERENCES
- Donahoe PK, Schnitzer JJ. Evaluation of the infant who has ambiguous genitalia, and principles of operative management. Semin Pediatr Surg. 1996;5(1):30-40.
- Gomes NL, Chetty T, Jorgensen A, Mitchell RT. Disorders of Sex Development-Novel Regulators, Impacts on Fertility, and Options for Fertility Preservation. Int J Mol Sci. 2020;21(7).
- Sreenivasan R, Gonen N, Sinclair A. SOX Genes and Their Role in Disorders of Sex Development. Sex Dev. 2022:1-12.
- Pask A. The Reproductive System. Adv Exp Med Biol. 2016;886:1-12.
- Tanaka SS, Nishinakamura R. Regulation of male sex determination: genital ridge formation and Sry activation in mice. Cell Mol Life Sci. 2014;71(24):4781-4802.
- Lucas-Herald AK, Bashamboo A. Gonadal development. Endocr Dev. 2014;27:1-16.
- Hughes IA, Houk C, Ahmed SF, Lee PA, Group LC, Group EC. Consensus statement on management of intersex disorders. Arch Dis Child. 2006;91(7):554-563.
- Lee PA, Houk CP, Ahmed SF, Hughes IA, Endocrinology ICCoIobtLWPESatESfP. Consensus statement on management of intersex disorders. International Consensus Conference on Intersex. Pediatrics. 2006;118(2):e488-500.
- Russell DW, Wilson JD. Chapter 4A - Steroid 5α-Reductase 2 Deficiency. In: Hammer MINLPTYWOMD, ed. Genetic Steroid Disorders. San Diego: Academic Press; 2014:199-214.
- Bennett NC, Gardiner RA, Hooper JD, Johnson DW, Gobe GC. Molecular cell biology of androgen receptor signalling. Int J Biochem Cell Biol. 2010;42(6):813-827.
- Wisniewski AB, Batista RL, Costa EMF, Finlayson C, Sircili MHP, Dénes FT, Domenice S, Mendonca BB. Management of 46,XY Differences/Disorders of Sex Development (DSD) Throughout Life. Endocr Rev. 2019.
- Mendonca BB, Domenice S, Arnhold IJ, Costa EM. 46,XY disorders of sex development (DSD). Clin Endocrinol (Oxf). 2009;70(2):173-187.
- Josso N, Picard JY, Rey R, di Clemente N. Testicular anti-Mullerian hormone: history, genetics, regulation and clinical applications. Pediatr Endocrinol Rev. 2006;3(4):347-358.
- Lahlou N, Roger M. Inhibin B in pubertal development and pubertal disorders. Semin Reprod Med.2004;22(3):165-175.
- Arnhold IJ, Mendonça BB, Diaz JA, Nogueira C, Batista MC, Madureira G, Oliveira D, Nicolau W, Bloise W. Prepubertal male pseudohermaphroditism due to 17-ketosteroid reductase deficiency: diagnostic value of a hCG test and lack of HLA association. J Endocrinol Invest. 1988;11(4):319-322.
- Guerra-Junior G, Andrade KC, Barcelos IHK, Maciel-Guerra AT. Imaging Techniques in the Diagnostic Journey of Disorders of Sex Development. Sex Dev. 2018;12(1-3):95-99.
- Garel L. Abnormal sex differentiation: who, how and when to image. Pediatr Radiol. 2008;38 Suppl 3:S508-511.
- Parivesh A, Barseghyan H, Délot E, Vilain E. Translating genomics to the clinical diagnosis of disorders/differences of sex development. Curr Top Dev Biol. 2019;134:317-375.
- Ahmed SF, Alimusina M, Batista RL, Domenice S, Lisboa Gomes N, McGowan R, Patjamontri S, Mendonca BB. The Use of Genetics for Reaching a Diagnosis in XY DSD. Sex Dev. 2022:1-18.
- Gomes NL, Batista RL, Nishi MY, Lerario AM, Silva TE, de Moraes Narcizo A, Benedetti AFF, de Assis Funari MF, Faria Junior JA, Moraes DR, Quintao LML, Montenegro LR, Ferrari MTM, Jorge AA, Arnhold IJP, Costa EMF, Domenice S, Mendonca BB. Contribution of Clinical and Genetic Approaches for Diagnosing 209 Index Cases With 46,XY Differences of Sex Development. J Clin Endocrinol Metab.2022;107(5):e1797-e1806.
- Igarashi M, Dung VC, Suzuki E, Ida S, Nakacho M, Nakabayashi K, Mizuno K, Hayashi Y, Kohri K, Kojima Y, Ogata T, Fukami M. Cryptic genomic rearrangements in three patients with 46,XY disorders of sex development. PLoS One. 2013;8(7):e68194.
- Kon M, Fukami M. Submicroscopic copy-number variations associated with 46,XY disorders of sex development. Mol Cell Pediatr. 2015;2(1):7.
- Ahmed SF, Hughes IA. The genetics of male undermasculinization. Clin Endocrinol (Oxf). 2002;56(1):1-18.
- Buonocore F, Achermann JC. Human sex development: targeted technologies to improve diagnosis. Genome Biol. 2016;17(1):257.
- Croft B, Ohnesorg T, Sinclair AH. The Role of Copy Number Variants in Disorders of Sex Development. Sex Dev. 2018;12(1-3):19-29.
- Eggers S, Sadedin S, van den Bergen JA, Robevska G, Ohnesorg T, Hewitt J, Lambeth L, Bouty A, Knarston IM, Tan TY, Cameron F, Werther G, Hutson J, O'Connell M, Grover SR, Heloury Y, Zacharin M, Bergman P, Kimber C, Brown J, Webb N, Hunter MF, Srinivasan S, Titmuss A, Verge CF, Mowat D, Smith G, Smith J, Ewans L, Shalhoub C, Crock P, Cowell C, Leong GM, Ono M, Lafferty AR, Huynh T, Visser U, Choong CS, McKenzie F, Pachter N, Thompson EM, Couper J, Baxendale A, Gecz J, Wheeler BJ, Jefferies C, MacKenzie K, Hofman P, Carter P, King RI, Krausz C, van Ravenswaaij-Arts CM, Looijenga L, Drop S, Riedl S, Cools M, Dawson A, Juniarto AZ, Khadilkar V, Khadilkar A, Bhatia V, Dũng VC, Atta I, Raza J, Thi Diem Chi N, Hao TK, Harley V, Koopman P, Warne G, Faradz S, Oshlack A, Ayers KL, Sinclair AH. Disorders of sex development: insights from targeted gene sequencing of a large international patient cohort. Genome Biol. 2016;17(1):243.
- Norling A, Linden Hirschberg A, Iwarsson E, Persson B, Wedell A, Barbaro M. Novel candidate genes for 46,XY gonadal dysgenesis identified by a customized 1 M array-CGH platform. Eur J Med Genet.2013;56(12):661-668.
- Ledig S, Hiort O, Scherer G, Hoffmann M, Wolff G, Morlot S, Kuechler A, Wieacker P. Array-CGH analysis in patients with syndromic and non-syndromic XY gonadal dysgenesis: evaluation of array CGH as diagnostic tool and search for new candidate loci. Hum Reprod. 2010;25(10):2637-2646.
- Parivesh A, Barseghyan H, Delot E, Vilain E. Translating genomics to the clinical diagnosis of disorders/differences of sex development. Curr Top Dev Biol. 2019;134:317-375.
- Dong Y, Yi Y, Yao H, Yang Z, Hu H, Liu J, Gao C, Zhang M, Zhou L, Asan, Yi X, Liang Z. Targeted next-generation sequencing identification of mutations in patients with disorders of sex development. BMC Med Genet. 2016;17:23.
- Bocher O, Genin E. Rare variant association testing in the non-coding genome. Hum Genet.2020;139(11):1345-1362.
- Ahmad-Nejad P, Ashavaid T, Vacaflores Salinas A, Huggett J, Harris K, Linder MW, Baluchova K, Steimer W, Payne DA, Diagnostics ICfM. Current and future challenges in quality assurance in molecular diagnostics. Clin Chim Acta. 2021;519:239-246.
- Jeong YH, Lu H, Park CH, Li M, Luo H, Kim JJ, Liu S, Ko KH, Huang S, Hwang IS, Kang MN, Gong D, Park KB, Choi EJ, Park JH, Jeong YW, Moon C, Hyun SH, Kim NH, Jeung EB, Yang H, Hwang WS, Gao F. Stochastic anomaly of methylome but persistent SRY hypermethylation in disorder of sex development in canine somatic cell nuclear transfer. Sci Rep. 2016;6:31088.
- Miyamoto N, Yoshida M, Kuratani S, Matsuo I, Aizawa S. Defects of urogenital development in mice lacking Emx2. Development. 1997;124(9):1653-1664.
- Wilhelm D, Englert C. The Wilms tumor suppressor WT1 regulates early gonad development by activation of Sf1. Genes Dev. 2002;16(14):1839-1851.
- Parker KL, Schedl A, Schimmer BP. Gene interactions in gonadal development. Annu Rev Physiol.1999;61:417-433.
- Parker KL, Schimmer BP, Schedl A. Genes essential for early events in gonadal development. Cell Mol Life Sci. 1999;55(6-7):831-838.
- Ito M, Yu R, Jameson JL. DAX-1 inhibits SF-1-mediated transactivation via a carboxy-terminal domain that is deleted in adrenal hypoplasia congenita. Mol Cell Biol. 1997;17(3):1476-1483.
- Hossain A, Saunders GF. The human sex-determining gene SRY is a direct target of WT1. J Biol Chem.2001;276(20):16817-16823.
- Tevosian SG, Albrecht KH, Crispino JD, Fujiwara Y, Eicher EM, Orkin SH. Gonadal differentiation, sex determination and normal Sry expression in mice require direct interaction between transcription partners GATA4 and FOG2. Development. 2002;129(19):4627-4634.
- Katoh-Fukui Y, Miyabayashi K, Komatsu T, Owaki A, Baba T, Shima Y, Kidokoro T, Kanai Y, Schedl A, Wilhelm D, Koopman P, Okuno Y, Morohashi K. Cbx2, a polycomb group gene, is required for Sry gene expression in mice. Endocrinology. 2012;153(2):913-924.
- Bashamboo A, Eozenou C, Rojo S, McElreavey K. Anomalies in human sex determination provide unique insights into the complex genetic interactions of early gonad development. Clin Genet.2017;91(2):143-156.
- Knower KC, Sim H, McClive PJ, Bowles J, Koopman P, Sinclair AH, Harley VR. Characterisation of urogenital ridge gene expression in the human embryonal carcinoma cell line NT2/D1. Sex Dev.2007;1(2):114-126.
- Bernard P, Sim H, Knower K, Vilain E, Harley V. Human SRY inhibits beta-catenin-mediated transcription. Int J Biochem Cell Biol. 2008;40(12):2889-2900.
- Kim Y, Kobayashi A, Sekido R, DiNapoli L, Brennan J, Chaboissier MC, Poulat F, Behringer RR, Lovell-Badge R, Capel B. Fgf9 and Wnt4 act as antagonistic signals to regulate mammalian sex determination. PLoS Biol. 2006;4(6):e187.
- Maatouk DM, DiNapoli L, Alvers A, Parker KL, Taketo MM, Capel B. Stabilization of beta-catenin in XY gonads causes male-to-female sex-reversal. Hum Mol Genet. 2008;17(19):2949-2955.
- Wilhelm D, Hiramatsu R, Mizusaki H, Widjaja L, Combes AN, Kanai Y, Koopman P. SOX9 regulates prostaglandin D synthase gene transcription in vivo to ensure testis development. J Biol Chem.2007;282(14):10553-10560.
- Moniot B, Declosmenil F, Barrionuevo F, Scherer G, Aritake K, Malki S, Marzi L, Cohen-Solal A, Georg I, Klattig J, Englert C, Kim Y, Capel B, Eguchi N, Urade Y, Boizet-Bonhoure B, Poulat F. The PGD2 pathway, independently of FGF9, amplifies SOX9 activity in Sertoli cells during male sexual differentiation. Development. 2009;136(11):1813-1821.
- Matson CK, Murphy MW, Sarver AL, Griswold MD, Bardwell VJ, Zarkower D. DMRT1 prevents female reprogramming in the postnatal mammalian testis. Nature. 2011;476(7358):101-104.
- Pearlman A, Loke J, Le Caignec C, White S, Chin L, Friedman A, Warr N, Willan J, Brauer D, Farmer C, Brooks E, Oddoux C, Riley B, Shajahan S, Camerino G, Homfray T, Crosby AH, Couper J, David A, Greenfield A, Sinclair A, Ostrer H. Mutations in MAP3K1 cause 46,XY disorders of sex development and implicate a common signal transduction pathway in human testis determination. Am J Hum Genet.2010;87(6):898-904.
- Loke J, Pearlman A, Radi O, Zuffardi O, Giussani U, Pallotta R, Camerino G, Ostrer H. Mutations in MAP3K1 tilt the balance from SOX9/FGF9 to WNT/beta-catenin signaling. Hum Mol Genet.2014;23(4):1073-1083.
- Bashamboo A, McElreavey K. Human sex-determination and disorders of sex-development (DSD). Semin Cell Dev Biol. 2015;45:77-83.
- Evilen da Silva T, Gomes NL, Lerário AM, Keegan CE, Nishi MY, Carvalho FM, Vilain E, Barseghyanm H, Martinez-Aguayo A, Forclaz MV, Papazian R, Pedroso de Paula LC, Costa EC, Carvalho LR, Jorge AA, Elias F, Mitchell R, Frade Costa EM, Mendonca BB, Domenice S. Genetic evidence of the association of DEAH-box helicase 37 defects with 46,XY gonadal dysgenesis spectrum. J Clin Endocrinol Metab. 2019.
- De Marchi M, Campagnoli C, Ghiringhello B, Ponzio G, Carbonara A. Gonadal agenesis in a phenotypically normal female with positive H-Y antigen. Hum Genet. 1981;56(3):417-419.
- Mendonca BB, Barbosa AS, Arnhold IJ, McElreavey K, Fellous M, Moreira-Filho CA. Gonadal agenesis in XX and XY sisters: evidence for the involvement of an autosomal gene. Am J Med Genet.1994;52(1):39-43.
- Ottolenghi C, Moreira-Filho C, Mendonca BB, Barbieri M, Fellous M, Berkovitz GD, McElreavey K. Absence of mutations involving the LIM homeobox domain gene LHX9 in 46,XY gonadal agenesis and dysgenesis. J Clin Endocrinol Metab. 2001;86(6):2465-2469.
- Swyer GI. Male pseudohermaphroditism: a hitherto undescribed form. Br Med J. 1955;2(4941):709-712.
- Josso N, Briard ML. Embryonic testicular regression syndrome: variable phenotypic expression in siblings. J Pediatr. 1980;97(2):200-204.
- Berkovitz GD, Fechner PY, Zacur HW, Rock JA, Snyder HM, 3rd, Migeon CJ, Perlman EJ. Clinical and pathologic spectrum of 46,XY gonadal dysgenesis: its relevance to the understanding of sex differentiation. Medicine (Baltimore). 1991;70(6):375-383.
- Viger RS, Guittot SM, Anttonen M, Wilson DB, Heikinheimo M. Role of the GATA family of transcription factors in endocrine development, function, and disease. Mol Endocrinol. 2008;22(4):781-798.
- Garg V, Kathiriya IS, Barnes R, Schluterman MK, King IN, Butler CA, Rothrock CR, Eapen RS, Hirayama-Yamada K, Joo K, Matsuoka R, Cohen JC, Srivastava D. GATA4 mutations cause human congenital heart defects and reveal an interaction with TBX5. Nature. 2003;424(6947):443-447.
- Wat MJ, Shchelochkov OA, Holder AM, Breman AM, Dagli A, Bacino C, Scaglia F, Zori RT, Cheung SW, Scott DA, Kang SH. Chromosome 8p23.1 deletions as a cause of complex congenital heart defects and diaphragmatic hernia. Am J Med Genet A. 2009;149A(8):1661-1677.
- Lourenco D, Brauner R, Rybczynska M, Nihoul-Fekete C, McElreavey K, Bashamboo A. Loss-of-function mutation in GATA4 causes anomalies of human testicular development. Proc Natl Acad Sci U S A. 2011;108(4):1597-1602.
- Finelli P, Pincelli AI, Russo S, Bonati MT, Recalcati MP, Masciadri M, Giardino D, Cavagnini F, Larizza L. Disruption of friend of GATA 2 gene (FOG-2) by a de novo t(8;10) chromosomal translocation is associated with heart defects and gonadal dysgenesis. Clin Genet. 2007;71(3):195-204.
- Bashamboo A, Brauner R, Bignon-Topalovic J, Lortat-Jacob S, Karageorgou V, Lourenco D, Guffanti A, McElreavey K. Mutations in the FOG2/ZFPM2 gene are associated with anomalies of human testis determination. Hum Mol Genet. 2014;23(14):3657-3665.
- van den Bergen JA, Robevska G, Eggers S, Riedl S, Grover SR, Bergman PB, Kimber C, Jiwane A, Khan S, Krausz C, Raza J, Atta I, Davis SR, Ono M, Harley V, Faradz SMH, Sinclair AH, Ayers KL. Analysis of variants in GATA4 and FOG2/ZFPM2 demonstrates benign contribution to 46,XY disorders of sex development. Mol Genet Genomic Med. 2020;8(3):e1095.
- Biason-Lauber A, Konrad D, Meyer M, DeBeaufort C, Schoenle EJ. Ovaries and female phenotype in a girl with 46,XY karyotype and mutations in the CBX2 gene. Am J Hum Genet. 2009;84(5):658-663.
- Sproll P, Eid W, Gomes CR, Mendonca BB, Gomes NL, Costa EM, Biason-Lauber A. Assembling the jigsaw puzzle: CBX2 isoform 2 and its targets in disorders/differences of sex development. Mol Genet Genomic Med. 2018;6(5):785-795.
- Elzaiat M, McElreavey K, Bashamboo A. Genetics of 46,XY gonadal dysgenesis. Best Pract Res Clin Endocrinol Metab. 2022;36(1):101633.
- Haber DA, Sohn RL, Buckler AJ, Pelletier J, Call KM, Housman DE. Alternative splicing and genomic structure of the Wilms tumor gene WT1. Proc Natl Acad Sci U S A. 1991;88(21):9618-9622.
- Melo KF, Martin RM, Costa EM, Carvalho FM, Jorge AA, Arnhold IJ, Mendonca BB. An unusual phenotype of Frasier syndrome due to IVS9 +4C>T mutation in the WT1 gene: predominantly male ambiguous genitalia and absence of gonadal dysgenesis. J Clin Endocrinol Metab. 2002;87(6):2500-2505.
- Ferrari MTM, Watanabe A, da Silva TE, Gomes NL, Batista RL, Nishi MY, de Paula LCP, Costa EC, Costa EMF, Cukier P, Onuchic LF, Mendonca BB, Domenice S. WT1 Pathogenic Variants are Associated with a Broad Spectrum of Differences in Sex Development Phenotypes and Heterogeneous Progression of Renal Disease. Sex Dev. 2022;16(1):46-54.
- Riccardi VM, Sujansky E, Smith AC, Francke U. Chromosomal imbalance in the Aniridia-Wilms' tumor association: 11p interstitial deletion. Pediatrics. 1978;61(4):604-610.
- van Heyningen V, Bickmore WA, Seawright A, Fletcher JM, Maule J, Fekete G, Gessler M, Bruns GA, Huerre-Jeanpierre C, Junien C, et al. Role for the Wilms tumor gene in genital development? Proc Natl Acad Sci U S A. 1990;87(14):5383-5386.
- Tiberio G, Digilio MC, Giannotti A. Obesity and WAGR syndrome. Clin Dysmorphol. 2000;9(1):63-64.
- Han JC, Liu QR, Jones M, Levinn RL, Menzie CM, Jefferson-George KS, Adler-Wailes DC, Sanford EL, Lacbawan FL, Uhl GR, Rennert OM, Yanovski JA. Brain-derived neurotrophic factor and obesity in the WAGR syndrome. N Engl J Med. 2008;359(9):918-927.
- Le Caignec C, Delnatte C, Vermeesch JR, Boceno M, Joubert M, Lavenant F, David A, Rival JM. Complete sex reversal in a WAGR syndrome patient. Am J Med Genet A. 2007;143A(22):2692-2695.
- Mueller RF. The Denys-Drash syndrome. J Med Genet. 1994;31(6):471-477.
- Baird PN, Santos A, Groves N, Jadresic L, Cowell JK. Constitutional mutations in the WT1 gene in patients with Denys-Drash syndrome. Hum Mol Genet. 1992;1(5):301-305.
- Pelletier J, Bruening W, Kashtan CE, Mauer SM, Manivel JC, Striegel JE, Houghton DC, Junien C, Habib R, Fouser L, et al. Germline mutations in the Wilms' tumor suppressor gene are associated with abnormal urogenital development in Denys-Drash syndrome. Cell. 1991;67(2):437-447.
- da Silva TE, Nishi MY, Costa EM, Martin RM, Carvalho FM, Mendonca BB, Domenice S. A novel WT1 heterozygous nonsense mutation (p.K248X) causing a mild and slightly progressive nephropathy in a 46,XY patient with Denys-Drash syndrome. Pediatr Nephrol. 2011;26(8):1311-1315.
- Gwin K, Cajaiba MM, Caminoa-Lizarralde A, Picazo ML, Nistal M, Reyes-Mugica M. Expanding the clinical spectrum of Frasier syndrome. Pediatr Dev Pathol. 2008;11(2):122-127.
- Drash A, Sherman F, Hartmann WH, Blizzard RM. A syndrome of pseudohermaphroditism, Wilms' tumor, hypertension, and degenerative renal disease. J Pediatr. 1970;76(4):585-593.
- Kohsaka T, Tagawa M, Takekoshi Y, Yanagisawa H, Tadokoro K, Yamada M. Exon 9 mutations in the WT1 gene, without influencing KTS splice isoforms, are also responsible for Frasier syndrome. Hum Mutat. 1999;14(6):466-470.
- Barbosa AS, Hadjiathanasiou CG, Theodoridis C, Papathanasiou A, Tar A, Merksz M, Gyorvari B, Sultan C, Dumas R, Jaubert F, Niaudet P, Moreira-Filho CA, Cotinot C, Fellous M. The same mutation affecting the splicing of WT1 gene is present on Frasier syndrome patients with or without Wilms' tumor. Hum Mutat. 1999;13(2):146-153.
- Morohashi K, Honda S, Inomata Y, Handa H, Omura T. A common trans-acting factor, Ad4-binding protein, to the promoters of steroidogenic P-450s. J Biol Chem. 1992;267(25):17913-17919.
- Lala DS, Rice DA, Parker KL. Steroidogenic factor I, a key regulator of steroidogenic enzyme expression, is the mouse homolog of fushi tarazu-factor I. Mol Endocrinol. 1992;6(8):1249-1258.
- Rice DA, Mouw AR, Bogerd AM, Parker KL. A shared promoter element regulates the expression of three steroidogenic enzymes. Mol Endocrinol. 1991;5(10):1552-1561.
- Lin L, Achermann JC. Steroidogenic factor-1 (SF-1, Ad4BP, NR5A1) and disorders of testis development. Sex Dev. 2008;2(4-5):200-209.
- Schimmer BP, White PC. Minireview: steroidogenic factor 1: its roles in differentiation, development, and disease. Mol Endocrinol. 2010;24(7):1322-1337.
- Majdic G, Young M, Gomez-Sanchez E, Anderson P, Szczepaniak LS, Dobbins RL, McGarry JD, Parker KL. Knockout mice lacking steroidogenic factor 1 are a novel genetic model of hypothalamic obesity. Endocrinology. 2002;143(2):607-614.
- Achermann JC, Ito M, Hindmarsh PC, Jameson JL. A mutation in the gene encoding steroidogenic factor-1 causes XY sex reversal and adrenal failure in humans. Nat Genet. 1999;22(2):125-126.
- Biason-Lauber A, Schoenle EJ. Apparently normal ovarian differentiation in a prepubertal girl with transcriptionally inactive steroidogenic factor 1 (NR5A1/SF-1) and adrenocortical insufficiency. Am J Hum Genet. 2000;67(6):1563-1568.
- Gerster K, Biason-Lauber A, Schoenle EJ. Clinical follow-up of the first SF-1 insufficient female patient. Ann Endocrinol (Paris). 2017;78(3):156-161.
- Achermann JC, Ozisik G, Ito M, Orun UA, Harmanci K, Gurakan B, Jameson JL. Gonadal determination and adrenal development are regulated by the orphan nuclear receptor steroidogenic factor-1, in a dose-dependent manner. J Clin Endocrinol Metab. 2002;87(4):1829-1833.
- Ferraz-de-Souza B, Lin L, Achermann JC. Steroidogenic factor-1 (SF-1, NR5A1) and human disease.Mol Cell Endocrinol. 2011;336(1-2):198-205.
- Lin L, Philibert P, Ferraz-de-Souza B, Kelberman D, Homfray T, Albanese A, Molini V, Sebire NJ, Einaudi S, Conway GS, Hughes IA, Jameson JL, Sultan C, Dattani MT, Achermann JC. Heterozygous missense mutations in steroidogenic factor 1 (SF1/Ad4BP, NR5A1) are associated with 46,XY disorders of sex development with normal adrenal function. J Clin Endocrinol Metab. 2007;92(3):991-999.
- Lourenco D, Brauner R, Lin L, De Perdigo A, Weryha G, Muresan M, Boudjenah R, Guerra-Junior G, Maciel-Guerra AT, Achermann JC, McElreavey K, Bashamboo A. Mutations in NR5A1 associated with ovarian insufficiency. N Engl J Med. 2009;360(12):1200-1210.
- Correa RV, Domenice S, Bingham NC, Billerbeck AE, Rainey WE, Parker KL, Mendonca BB. A microdeletion in the ligand binding domain of human steroidogenic factor 1 causes XY sex reversal without adrenal insufficiency. J Clin Endocrinol Metab. 2004;89(4):1767-1772.
- Urs AN, Dammer E, Sewer MB. Sphingosine regulates the transcription of CYP17 by binding to steroidogenic factor-1. Endocrinology. 2006;147(11):5249-5258.
- Domenice S, Zamboni Machado A, Moraes Ferreira F, Ferraz-de-Souza B, Marcondes Lerario A, Lin L, Yumie Nishi M, Lisboa Gomes N, Evelin da Silva T, Barbosa Silva R, Vieira Correa R, Ribeiro Montenegro L, Narciso A, Maria Frade Costa E, C Achermann J, Bilharinho Mendonca B. Wide spectrum of NR5A1-related phenotypes in 46,XY and 46,XX individuals. Birth Defects Res C Embryo Today. 2016;108(4):309-320.
- Fabbri HC, Ribeiro de Andrade JG, Maciel-Guerra AT, Guerra-Júnior G, de Mello MP. NR5A1 Loss-of-Function Mutations Lead to 46,XY Partial Gonadal Dysgenesis Phenotype: Report of Three Novel Mutations. Sex Dev. 2016;10(4):191-199.
- Fabbri-Scallet H, de Sousa LM, Maciel-Guerra AT, Guerra-Júnior G, de Mello MP. Mutation update for the NR5A1 gene involved in DSD and infertility. Hum Mutat. 2020;41(1):58-68.
- Pedace L, Laino L, Preziosi N, Valentini MS, Scommegna S, Rapone AM, Guarino N, Boscherini B, De Bernardo C, Marrocco G, Majore S, Grammatico P. Longitudinal hormonal evaluation in a patient with disorder of sexual development, 46,XY karyotype and one NR5A1 mutation. Am J Med Genet A.2014;164A(11):2938-2946.
- Tantawy S, Mazen I, Soliman H, Anwar G, Atef A, El-Gammal M, El-Kotoury A, Mekkawy M, Torky A, Rudolf A, Schrumpf P, Grüters A, Krude H, Dumargne MC, Astudillo R, Bashamboo A, Biebermann H, Köhler B. Analysis of the gene coding for steroidogenic factor 1 (SF1, NR5A1) in a cohort of 50 Egyptian patients with 46,XY disorders of sex development. Eur J Endocrinol. 2014;170(5):759-767.
- Warman DM, Costanzo M, Marino R, Berensztein E, Galeano J, Ramirez PC, Saraco N, Baquedano MS, Ciaccio M, Guercio G, Chaler E, Maceiras M, Lazzatti JM, Bailez M, Rivarola MA, Belgorosky A. Three new SF-1 (NR5A1) gene mutations in two unrelated families with multiple affected members: within-family variability in 46,XY subjects and low ovarian reserve in fertile 46,XX subjects. Horm Res Paediatr. 2011;75(1):70-77.
- Mazen I, Abdel-Hamid M, Mekkawy M, Bignon-Topalovic J, Boudjenah R, El Gammal M, Essawi M, Bashamboo A, McElreavey K. Identification of NR5A1 Mutations and Possible Digenic Inheritance in 46,XY Gonadal Dysgenesis. Sex Dev. 2016;10(3):147-151.
- Allali S, Muller JB, Brauner R, Lourenco D, Boudjenah R, Karageorgou V, Trivin C, Lottmann H, Lortat-Jacob S, Nihoul-Fekete C, De Dreuzy O, McElreavey K, Bashamboo A. Mutation analysis of NR5A1 encoding steroidogenic factor 1 in 77 patients with 46, XY disorders of sex development (DSD) including hypospadias. PLoS One. 2011;6(10):e24117.
- Bashamboo A, Ferraz-de-Souza B, Lourenço D, Lin L, Sebire NJ, Montjean D, Bignon-Topalovic J, Mandelbaum J, Siffroi JP, Christin-Maitre S, Radhakrishna U, Rouba H, Ravel C, Seeler J, Achermann JC, McElreavey K. Human male infertility associated with mutations in NR5A1 encoding steroidogenic factor 1. Am J Hum Genet. 2010;87(4):505-512.
- El-Khairi R, Achermann JC. Steroidogenic factor-1 and human disease. Semin Reprod Med.2012;30(5):374-381.
- Tantawy S, Lin L, Akkurt I, Borck G, Klingmuller D, Hauffa BP, Krude H, Biebermann H, Achermann JC, Kohler B. Testosterone production during puberty in two 46,XY patients with disorders of sex development and novel NR5A1 (SF-1) mutations. Eur J Endocrinol. 2012;167(1):125-130.
- Philibert P, Zenaty D, Lin L, Soskin S, Audran F, Léger J, Achermann JC, Sultan C. Mutational analysis of steroidogenic factor 1 (NR5a1) in 24 boys with bilateral anorchia: a French collaborative study. Hum Reprod. 2007;22(12):3255-3261.
- Camats N, Pandey AV, Fernandez-Cancio M, Andaluz P, Janner M, Toran N, Moreno F, Bereket A, Akcay T, Garcia-Garcia E, Munoz MT, Gracia R, Nistal M, Castano L, Mullis PE, Carrascosa A, Audi L, Fluck CE. Ten novel mutations in the NR5A1 gene cause disordered sex development in 46,XY and ovarian insufficiency in 46,XX individuals. J Clin Endocrinol Metab. 2012;97(7):E1294-1306.
- Smith AM RN, Robin NH. NR5A1 Pathogenic Variant Identified in Non-Syndromic 46, XY Ovotesticular Disorder of Sexual Development. Archives of Pediatrics. 2019;4(162. Knarston IM, Robevska G, van den Bergen JA, Eggers S, Croft B, Yates J, Hersmus R, Looijenga LHJ, Cameron FJ, Monhike K, Ayers KL, Sinclair AH. NR5A1 gene variants repress the ovarian-specific WNT signaling pathway in 46,XX disorders of sex development patients. Hum Mutat. 2019;40(2):207-216.
- Vilain E, Elreavey KM, Richaud F, Fellous M. [Sex genetics]. Presse Med. 1992;21(18):852-856.
- Hawkins JR. Mutational analysis of SRY in XY females. Hum Mutat. 1993;2(5):347-350.
- McElreavey K, Vilain E, Barbaux S, Fuqua JS, Fechner PY, Souleyreau N, Doco-Fenzy M, Gabriel R, Quereux C, Fellous M, Berkovitz GD. Loss of sequences 3' to the testis-determining gene, SRY, including the Y pseudoautosomal boundary associated with partial testicular determination. Proc Natl Acad Sci U S A. 1996;93(16):8590-8594.
- Harley VR, Jackson DI, Hextall PJ, Hawkins JR, Berkovitz GD, Sockanathan S, Lovell-Badge R, Goodfellow PN. DNA binding activity of recombinant SRY from normal males and XY females. Science.1992;255(5043):453-456.
- Schmitt-Ney M, Thiele H, Kaltwasser P, Bardoni B, Cisternino M, Scherer G. Two novel SRY missense mutations reducing DNA binding identified in XY females and their mosaic fathers. Am J Hum Genet.1995;56(4):862-869.
- Assumpcao JG, Benedetti CE, Maciel-Guerra AT, Guerra G, Jr., Baptista MT, Scolfaro MR, de Mello MP. Novel mutations affecting SRY DNA-binding activity: the HMG box N65H associated with 46,XY pure gonadal dysgenesis and the familial non-HMG box R30I associated with variable phenotypes. J Mol Med (Berl). 2002;80(12):782-790.
- Foster JW, Dominguez-Steglich MA, Guioli S, Kwok C, Weller PA, Stevanovic M, Weissenbach J, Mansour S, Young ID, Goodfellow PN, et al. Campomelic dysplasia and autosomal sex reversal caused by mutations in an SRY-related gene. Nature. 1994;372(6506):525-530.
- Wagner T, Wirth J, Meyer J, Zabel B, Held M, Zimmer J, Pasantes J, Bricarelli FD, Keutel J, Hustert E, Wolf U, Tommerup N, Schempp W, Scherer G. Autosomal sex reversal and campomelic dysplasia are caused by mutations in and around the SRY-related gene SOX9. Cell. 1994;79(6):1111-1120.
- Kwok C, Weller PA, Guioli S, Foster JW, Mansour S, Zuffardi O, Punnett HH, Dominguez-Steglich MA, Brook JD, Young ID, et al. Mutations in SOX9, the gene responsible for Campomelic dysplasia and autosomal sex reversal. Am J Hum Genet. 1995;57(5):1028-1036.
- Croft B, Ohnesorg T, Hewitt J, Bowles J, Quinn A, Tan J, Corbin V, Pelosi E, van den Bergen J, Sreenivasan R, Knarston I, Robevska G, Vu DC, Hutson J, Harley V, Ayers K, Koopman P, Sinclair A. Human sex reversal is caused by duplication or deletion of core enhancers upstream of SOX9. Nat Commun. 2018;9(1):5319.
- Migale R, Neumann M, Lovell-Badge R. Long-Range Regulation of Key Sex Determination Genes. Sex Dev. 2021;15(5-6):360-380.
- Pop R, Conz C, Lindenberg KS, Blesson S, Schmalenberger B, Briault S, Pfeifer D, Scherer G. Screening of the 1 Mb SOX9 5' control region by array CGH identifies a large deletion in a case of campomelic dysplasia with XY sex reversal. J Med Genet. 2004;41(4):e47.
- Velagaleti GV, Bien-Willner GA, Northup JK, Lockhart LH, Hawkins JC, Jalal SM, Withers M, Lupski JR, Stankiewicz P. Position effects due to chromosome breakpoints that map approximately 900 Kb upstream and approximately 1.3 Mb downstream of SOX9 in two patients with campomelic dysplasia. Am J Hum Genet. 2005;76(4):652-662.
- Bagheri-Fam S, Sim H, Bernard P, Jayakody I, Taketo MM, Scherer G, Harley VR. Loss of Fgfr2 leads to partial XY sex reversal. Dev Biol. 2008;314(1):71-83.
- Colvin JS, Green RP, Schmahl J, Capel B, Ornitz DM. Male-to-female sex reversal in mice lacking fibroblast growth factor 9. Cell. 2001;104(6):875-889.
- Jameson SA, Lin YT, Capel B. Testis development requires the repression of Wnt4 by Fgf signaling. Dev Biol. 2012;370(1):24-32.
- Kim Y, Bingham N, Sekido R, Parker KL, Lovell-Badge R, Capel B. Fibroblast growth factor receptor 2 regulates proliferation and Sertoli differentiation during male sex determination. Proc Natl Acad Sci U S A. 2007;104(42):16558-16563.
- Machado AZ, da Silva TE, Frade Costa EM, Dos Santos MG, Nishi MY, Brito VN, Mendonca BB, Domenice S. Absence of inactivating mutations and deletions in the DMRT1 and FGF9 genes in a large cohort of 46,XY patients with gonadal dysgenesis. Eur J Med Genet. 2012;55(12):690-694.
- Reardon W, Winter RM, Rutland P, Pulleyn LJ, Jones BM, Malcolm S. Mutations in the fibroblast growth factor receptor 2 gene cause Crouzon syndrome. Nat Genet. 1994;8(1):98-103.
- Kan SH, Elanko N, Johnson D, Cornejo-Roldan L, Cook J, Reich EW, Tomkins S, Verloes A, Twigg SR, Rannan-Eliya S, McDonald-McGinn DM, Zackai EH, Wall SA, Muenke M, Wilkie AO. Genomic screening of fibroblast growth-factor receptor 2 reveals a wide spectrum of mutations in patients with syndromic craniosynostosis. Am J Hum Genet. 2002;70(2):472-486.
- Roscioli T, Elakis G, Cox TC, Moon DJ, Venselaar H, Turner AM, Le T, Hackett E, Haan E, Colley A, Mowat D, Worgan L, Kirk EP, Sachdev R, Thompson E, Gabbett M, McGaughran J, Gibson K, Gattas M, Freckmann ML, Dixon J, Hoefsloot L, Field M, Hackett A, Kamien B, Edwards M, Ades LC, Collins FA, Wilson MJ, Savarirayan R, Tan TY, Amor DJ, McGillivray G, White SM, Glass IA, David DJ, Anderson PJ, Gianoutsos M, Buckley MF. Genotype and clinical care correlations in craniosynostosis: findings from a cohort of 630 Australian and New Zealand patients. Am J Med Genet C Semin Med Genet. 2013;163C(4):259-270.
- Shams I, Rohmann E, Eswarakumar VP, Lew ED, Yuzawa S, Wollnik B, Schlessinger J, Lax I. Lacrimo-auriculo-dento-digital syndrome is caused by reduced activity of the fibroblast growth factor 10 (FGF10)-FGF receptor 2 signaling pathway. Mol Cell Biol. 2007;27(19):6903-6912.
- Bagheri-Fam S, Ono M, Li L, Zhao L, Ryan J, Lai R, Katsura Y, Rossello FJ, Koopman P, Scherer G, Bartsch O, Eswarakumar JV, Harley VR. FGFR2 mutation in 46,XY sex reversal with craniosynostosis. Hum Mol Genet. 2015;24(23):6699-6710.
- Tate G, Satoh H, Endo Y, Mitsuya T. Assignment of desert hedgehog (DHH) to human chromosome bands 12q12-->q13.1 by in situ hybridization. Cytogenet Cell Genet. 2000;88(1-2):93-94.
- Yao HH, Whoriskey W, Capel B. Desert Hedgehog/Patched 1 signaling specifies fetal Leydig cell fate in testis organogenesis. Genes Dev. 2002;16(11):1433-1440.
- Umehara F, Tate G, Itoh K, Yamaguchi N, Douchi T, Mitsuya T, Osame M. A novel mutation of desert hedgehog in a patient with 46,XY partial gonadal dysgenesis accompanied by minifascicular neuropathy. Am J Hum Genet. 2000;67(5):1302-1305.
- Canto P, Soderlund D, Reyes E, Mendez JP. Mutations in the desert hedgehog (DHH) gene in patients with 46,XY complete pure gonadal dysgenesis. J Clin Endocrinol Metab. 2004;89(9):4480-4483.
- Canto P, Vilchis F, Soderlund D, Reyes E, Mendez JP. A heterozygous mutation in the desert hedgehog gene in patients with mixed gonadal dysgenesis. Mol Hum Reprod. 2005;11(11):833-836.
- Das DK, Sanghavi D, Gawde H, Idicula-Thomas S, Vasudevan L. Novel homozygous mutations in Desert hedgehog gene in patients with 46,XY complete gonadal dysgenesis and prediction of its structural and functional implications by computational methods. Eur J Med Genet. 2011;54(6):e529-534.
- Werner R, Merz H, Birnbaum W, Marshall L, Schroder T, Reiz B, Kavran JM, Baumer T, Capetian P, Hiort O. 46,XY Gonadal Dysgenesis due to a Homozygous Mutation in Desert Hedgehog (DHH) Identified by Exome Sequencing. J Clin Endocrinol Metab. 2015;100(7):E1022-1029.
- Callier P, Calvel P, Matevossian A, Makrythanasis P, Bernard P, Kurosaka H, Vannier A, Thauvin-Robinet C, Borel C, Mazaud-Guittot S, Rolland A, Desdoits-Lethimonier C, Guipponi M, Zimmermann C, Stévant I, Kuhne F, Conne B, Santoni F, Lambert S, Huet F, Mugneret F, Jaruzelska J, Faivre L, Wilhelm D, Jégou B, Trainor PA, Resh MD, Antonarakis SE, Nef S. Loss of function mutation in the palmitoyl-transferase HHAT leads to syndromic 46,XY disorder of sex development by impeding Hedgehog protein palmitoylation and signaling. PLoS Genet. 2014;10(5):e1004340.
- Agha Z, Iqbal Z, Azam M, Ayub H, Vissers LE, Gilissen C, Ali SH, Riaz M, Veltman JA, Pfundt R, van Bokhoven H, Qamar R. Exome sequencing identifies three novel candidate genes implicated in intellectual disability. PLoS One. 2014;9(11):e112687.
- Raymond CS, Parker ED, Kettlewell JR, Brown LG, Page DC, Kusz K, Jaruzelska J, Reinberg Y, Flejter WL, Bardwell VJ, Hirsch B, Zarkower D. A region of human chromosome 9p required for testis development contains two genes related to known sexual regulators. Hum Mol Genet. 1999;8(6):989-996.
- Raymond CS, Murphy MW, O'Sullivan MG, Bardwell VJ, Zarkower D. Dmrt1, a gene related to worm and fly sexual regulators, is required for mammalian testis differentiation. Genes Dev.2000;14(20):2587-2595.
- Raymond CS, Shamu CE, Shen MM, Seifert KJ, Hirsch B, Hodgkin J, Zarkower D. Evidence for evolutionary conservation of sex-determining genes. Nature. 1998;391(6668):691-695.
- Muroya K, Okuyama T, Goishi K, Ogiso Y, Fukuda S, Kameyama J, Sato H, Suzuki Y, Terasaki H, Gomyo H, Wakui K, Fukushima Y, Ogata T. Sex-determining gene(s) on distal 9p: clinical and molecular studies in six cases. J Clin Endocrinol Metab. 2000;85(9):3094-3100.
- Buonocore F, Clifford-Mobley O, King TFJ, Striglioni N, Man E, Suntharalingham JP, Del Valle I, Lin L, Lagos CF, Rumsby G, Conway GS, Achermann JC. Next-Generation Sequencing Reveals Novel Genetic Variants (SRY, DMRT1, NR5A1, DHH, DHX37) in Adults With 46,XY DSD. J Endocr Soc.2019;3(12):2341-2360.
- Zarkower D, Murphy MW. DMRT1: An Ancient Sexual Regulator Required for Human Gonadogenesis. Sex Dev. 2021:1-14.
- Gibbons RJ, Higgs DR. Molecular-clinical spectrum of the ATR-X syndrome. Am J Med Genet.2000;97(3):204-212.
- Wilkie AO, Gibbons RJ, Higgs DR, Pembrey ME. X linked alpha thalassaemia/mental retardation: spectrum of clinical features in three related males. J Med Genet. 1991;28(11):738-741.
- Linhares ND, Valadares ER, da Costa SS, Arantes RR, de Oliveira LR, Rosenberg C, Vianna-Morgante AM, Svartman M. Inherited Xq13.2-q21.31 duplication in a boy with recurrent seizures and pubertal gynecomastia: Clinical, chromosomal and aCGH characterization. Meta Gene. 2016;9:185-190.
- Badens C, Lacoste C, Philip N, Martini N, Courrier S, Giuliano F, Verloes A, Munnich A, Leheup B, Burglen L, Odent S, Van Esch H, Levy N. Mutations in PHD-like domain of the ATRX gene correlate with severe psychomotor impairment and severe urogenital abnormalities in patients with ATRX syndrome. Clin Genet. 2006;70(1):57-62.
- Gibbons RJ, Wada T, Fisher CA, Malik N, Mitson MJ, Steensma DP, Fryer A, Goudie DR, Krantz ID, Traeger-Synodinos J. Mutations in the chromatin-associated protein ATRX. Hum Mutat. 2008;29(6):796-802.
- Tang P, Park DJ, Marshall Graves JA, Harley VR. ATRX and sex differentiation. Trends Endocrinol Metab. 2004;15(7):339-344.
- Bogani D, Siggers P, Brixey R, Warr N, Beddow S, Edwards J, Williams D, Wilhelm D, Koopman P, Flavell RA, Chi H, Ostrer H, Wells S, Cheeseman M, Greenfield A. Loss of mitogen-activated protein kinase kinase kinase 4 (MAP3K4) reveals a requirement for MAPK signalling in mouse sex determination. PLoS Biol. 2009;7(9):e1000196.
- Gierl MS, Gruhn WH, von Seggern A, Maltry N, Niehrs C. GADD45G functions in male sex determination by promoting p38 signaling and Sry expression. Dev Cell. 2012;23(5):1032-1042.
- Warr N, Bogani D, Siggers P, Brixey R, Tateossian H, Dopplapudi A, Wells S, Cheeseman M, Xia Y, Ostrer H, Greenfield A. Minor abnormalities of testis development in mice lacking the gene encoding the MAPK signalling component, MAP3K1. PLoS One. 2011;6(5):e19572.
- Charlaftis N, Suddason T, Wu X, Anwar S, Karin M, Gallagher E. The MEKK1 PHD ubiquitinates TAB1 to activate MAPKs in response to cytokines. EMBO J. 2014;33(21):2581-2596.
- Bardoni B, Zanaria E, Guioli S, Floridia G, Worley KC, Tonini G, Ferrante E, Chiumello G, McCabe ER, Fraccaro M, et al. A dosage sensitive locus at chromosome Xp21 is involved in male to female sex reversal. Nat Genet. 1994;7(4):497-501.
- Sanlaville D, Vialard F, Thepot F, Vue-Droy L, Ardalan A, Nizard P, Corre A, Devauchelle B, Martin-Denavit T, Nouchy M, Malan V, Taillemite JL, Portnoi MF. Functional disomy of Xp including duplication of DAX1 gene with sex reversal due to t(X;Y)(p21.2;p11.3). Am J Med Genet A. 2004;128A(3):325-330.
- Moyses-Oliveira M, Guilherme RS, Meloni VA, Di Battista A, de Mello CB, Bragagnolo S, Moretti-Ferreira D, Kosyakova N, Liehr T, Carvalheira GM, Melaragno MI. X-linked intellectual disability related genes disrupted by balanced X-autosome translocations. Am J Med Genet B Neuropsychiatr Genet.2015;168(8):669-677.
- Carrie A, Jun L, Bienvenu T, Vinet MC, McDonell N, Couvert P, Zemni R, Cardona A, Van Buggenhout G, Frints S, Hamel B, Moraine C, Ropers HH, Strom T, Howell GR, Whittaker A, Ross MT, Kahn A, Fryns JP, Beldjord C, Marynen P, Chelly J. A new member of the IL-1 receptor family highly expressed in hippocampus and involved in X-linked mental retardation. Nat Genet. 1999;23(1):25-31.
- Barbaro M, Oscarson M, Schoumans J, Staaf J, Ivarsson SA, Wedell A. Isolated 46,XY gonadal dysgenesis in two sisters caused by a Xp21.2 interstitial duplication containing the DAX1 gene. J Clin Endocrinol Metab. 2007;92(8):3305-3313.
- Barbaro M, Cicognani A, Balsamo A, Lofgren A, Baldazzi L, Wedell A, Oscarson M. Gene dosage imbalances in patients with 46,XY gonadal DSD detected by an in-house-designed synthetic probe set for multiplex ligation-dependent probe amplification analysis. Clin Genet. 2008;73(5):453-464.
- Smyk M, Berg JS, Pursley A, Curtis FK, Fernandez BA, Bien-Willner GA, Lupski JR, Cheung SW, Stankiewicz P. Male-to-female sex reversal associated with an approximately 250 kb deletion upstream of NR0B1 (DAX1). Hum Genet. 2007;122(1):63-70.
- White S, Ohnesorg T, Notini A, Roeszler K, Hewitt J, Daggag H, Smith C, Turbitt E, Gustin S, van den Bergen J, Miles D, Western P, Arboleda V, Schumacher V, Gordon L, Bell K, Bengtsson H, Speed T, Hutson J, Warne G, Harley V, Koopman P, Vilain E, Sinclair A. Copy number variation in patients with disorders of sex development due to 46,XY gonadal dysgenesis. PLoS One. 2011;6(3):e17793.
- Stark K, Vainio S, Vassileva G, McMahon AP. Epithelial transformation of metanephric mesenchyme in the developing kidney regulated by Wnt-4. Nature. 1994;372(6507):679-683.
- Elejalde BR, Opitz JM, de Elejalde MM, Gilbert EF, Abellera M, Meisner L, Lebel RR, Hartigan JM. Tandem dup (1p) within the short arm of chromosome 1 in a child with ambiguous genitalia and multiple congenital anomalies. Am J Med Genet. 1984;17(4):723-730.
- Jordan BK, Mohammed M, Ching ST, Delot E, Chen XN, Dewing P, Swain A, Rao PN, Elejalde BR, Vilain E. Up-regulation of WNT-4 signaling and dosage-sensitive sex reversal in humans. Am J Hum Genet. 2001;68(5):1102-1109.
- McElreavey K, Jorgensen A, Eozenou C, Merel T, Bignon-Topalovic J, Tan DS, Houzelstein D, Buonocore F, Warr N, Kay RGG, Peycelon M, Siffroi JP, Mazen I, Achermann JC, Shcherbak Y, Leger J, Sallai A, Carel JC, Martinerie L, Le Ru R, Conway GS, Mignot B, Van Maldergem L, Bertalan R, Globa E, Brauner R, Jauch R, Nef S, Greenfield A, Bashamboo A. Pathogenic variants in the DEAH-box RNA helicase DHX37 are a frequent cause of 46,XY gonadal dysgenesis and 46,XY testicular regression syndrome. Genet Med. 2020;22(1):150-159.
- Le Caignec C, Baron S, McElreavey K, Joubert M, Rival JM, Mechinaud F, David A. 46,XY gonadal dysgenesis: evidence for autosomal dominant transmission in a large kindred. Am J Med Genet A.2003;116A(1):37-43.
- Fechner PY, Marcantonio SM, Ogata T, Rosales TO, Smith KD, Goodfellow PN, Migeon CJ, Berkovitz GD. Report of a kindred with X-linked (or autosomal dominant sex-limited) 46,XY partial gonadal dysgenesis. J Clin Endocrinol Metab. 1993;76(5):1248-1253.
- Ostrer H. Pathogenic Variants in MAP3K1 Cause 46,XY Gonadal Dysgenesis: A Review. Sex Dev.2022:1-6.
- Opitz JM. RSH/SLO ("Smith-Lemli-Opitz") syndrome: historical, genetic, and developmental considerations. Am J Med Genet. 1994;50(4):344-346.
- Tint GS, Irons M, Elias ER, Batta AK, Frieden R, Chen TS, Salen G. Defective cholesterol biosynthesis associated with the Smith-Lemli-Opitz syndrome. N Engl J Med. 1994;330(2):107-113.
- Fukazawa R, Nakahori Y, Kogo T, Kawakami T, Akamatsu H, Tanae A, Hibi I, Nagafuchi S, Nakagome Y, Hirayama T. Normal Y sequences in Smith-Lemli-Opitz syndrome with total failure of masculinization. Acta Paediatr. 1992;81(6-7):570-572.
- Joseph DB, Uehling DT, Gilbert E, Laxova R. Genitourinary abnormalities associated with the Smith-Lemli-Opitz syndrome. J Urol. 1987;137(4):719-721.
- Bianconi SE, Cross JL, Wassif CA, Porter FD. Pathogenesis, Epidemiology, Diagnosis and Clinical Aspects of Smith-Lemli-Opitz Syndrome. Expert Opin Orphan Drugs. 2015;3(3):267-280.
- Andersson HC, Frentz J, Martinez JE, Tuck-Muller CM, Bellizaire J. Adrenal insufficiency in Smith-Lemli-Opitz syndrome. Am J Med Genet. 1999;82(5):382-384.
- Bianconi SE, Conley SK, Keil MF, Sinaii N, Rother KI, Porter FD, Stratakis CA. Adrenal function in Smith-Lemli-Opitz syndrome. Am J Med Genet A. 2011;155A(11):2732-2738.
- Correa-Cerro LS, Porter FD. 3beta-hydroxysterol Delta7-reductase and the Smith-Lemli-Opitz syndrome. Mol Genet Metab. 2005;84(2):112-126.
- Porter FD. Smith-Lemli-Opitz syndrome: pathogenesis, diagnosis and management. Eur J Hum Genet.2008;16(5):535-541.
- Correa-Cerro LS, Wassif CA, Waye JS, Krakowiak PA, Cozma D, Dobson NR, Levin SW, Anadiotis G, Steiner RD, Krajewska-Walasek M, Nowaczyk MJ, Porter FD. DHCR7 nonsense mutations and characterisation of mRNA nonsense mediated decay in Smith-Lemli-Opitz syndrome. J Med Genet.2005;42(4):350-357.
- Tierney E, Nwokoro NA, Porter FD, Freund LS, Ghuman JK, Kelley RI. Behavior phenotype in the RSH/Smith-Lemli-Opitz syndrome. Am J Med Genet. 2001;98(2):191-200.
- Sikora DM, Ruggiero M, Petit-Kekel K, Merkens LS, Connor WE, Steiner RD. Cholesterol supplementation does not improve developmental progress in Smith-Lemli-Opitz syndrome. J Pediatr.2004;144(6):783-791.
- Jira PE, Wevers RA, de Jong J, Rubio-Gozalbo E, Janssen-Zijlstra FS, van Heyst AF, Sengers RC, Smeitink JA. Simvastatin. A new therapeutic approach for Smith-Lemli-Opitz syndrome. J Lipid Res.2000;41(8):1339-1346.
- Wassif CA, Kratz L, Sparks SE, Wheeler C, Bianconi S, Gropman A, Calis KA, Kelley RI, Tierney E, Porter FD. A placebo-controlled trial of simvastatin therapy in Smith-Lemli-Opitz syndrome. Genet Med.2017;19(3):297-305.
- Pasta S, Akhile O, Tabron D, Ting F, Shackleton C, Watson G. Delivery of the 7-dehydrocholesterol reductase gene to the central nervous system using adeno-associated virus vector in a mouse model of Smith-Lemli-Opitz Syndrome. Mol Genet Metab Rep. 2015;4:92-98.
- Misrahi M, Meduri G, Pissard S, Bouvattier C, Beau I, Loosfelt H, Jolivet A, Rappaport R, Milgrom E, Bougneres P. Comparison of immunocytochemical and molecular features with the phenotype in a case of incomplete male pseudohermaphroditism associated with a mutation of the luteinizing hormone receptor. J Clin Endocrinol Metab. 1997;82(7):2159-2165.
- Martens JW, Verhoef-Post M, Abelin N, Ezabella M, Toledo SP, Brunner HG, Themmen AP. A homozygous mutation in the luteinizing hormone receptor causes partial Leydig cell hypoplasia: correlation between receptor activity and phenotype. Mol Endocrinol. 1998;12(6):775-784.
- Toledo SP, Arnhold IJ, Luthold W, Russo EM, Saldanha PH. Leydig cell hypoplasia determining familial hypergonadotropic hypogonadism. Prog Clin Biol Res. 1985;200:311-314.
- Zenteno JC, Canto P, Kofman-Alfaro S, Mendez JP. Evidence for genetic heterogeneity in male pseudohermaphroditism due to Leydig cell hypoplasia. J Clin Endocrinol Metab. 1999;84(10):3803-3806.
- Arnhold IJ, de Mendonca BB, Toledo SP, Madureira G, Nicolau W, Bisi H, Bloise W. Leydig cell hypoplasia causing male pseudohermaphroditism: case report and review of the literature. Rev Hosp Clin Fac Med Sao Paulo. 1987;42(5):227-232.
- Gromoll J, Eiholzer U, Nieschlag E, Simoni M. Male hypogonadism caused by homozygous deletion of exon 10 of the luteinizing hormone (LH) receptor: differential action of human chorionic gonadotropin and LH. J Clin Endocrinol Metab. 2000;85(6):2281-2286.
- Richard N, Leprince C, Gruchy N, Pigny P, Andrieux J, Mittre H, Manouvrier S, Lahlou N, Weill J, Kottler ML. Identification by array-Comparative Genomic Hybridization (array-CGH) of a large deletion of luteinizing hormone receptor gene combined with a missense mutation in a patient diagnosed with a 46,XY disorder of sex development and application to prenatal diagnosis. Endocr J. 2011;58(9):769-776.
- Stavrou SS, Zhu YS, Cai LQ, Katz MD, Herrera C, Defillo-Ricart M, Imperato-McGinley J. A novel mutation of the human luteinizing hormone receptor in 46XY and 46XX sisters. J Clin Endocrinol Metab.1998;83(6):2091-2098.
- Qiao J, Han B, Liu BL, Chen X, Ru Y, Cheng KX, Chen FG, Zhao SX, Liang J, Lu YL, Tang JF, Wu YX, Wu WL, Chen JL, Chen MD, Song HD. A splice site mutation combined with a novel missense mutation of LHCGR cause male pseudohermaphroditism. Hum Mutat. 2009;30(9):E855-865.
- Zhou HX. Effect of mixed macromolecular crowding agents on protein folding. Proteins.2008;72(4):1109-1113.
- Yariz KO, Walsh T, Uzak A, Spiliopoulos M, Duman D, Onalan G, King MC, Tekin M. Inherited mutation of the luteinizing hormone/choriogonadotropin receptor (LHCGR) in empty follicle syndrome. Fertil Steril. 2011;96(2):e125-130.
- Aktar Karakaya A, Çayır A, Unal E, Beştaş A, Ece Solmaz A, Kenan Haspolat Y. A rare cause of primary amenorrhea: LHCGR gene mutations. Eur J Obstet Gynecol Reprod Biol. 2022;272:193-197.
- Latronico AC, Arnhold IJ. Inactivating mutations of the human luteinizing hormone receptor in both sexes. Semin Reprod Med. 2012;30(5):382-386.
- Latronico AC, Anasti J, Arnhold IJ, Rapaport R, Mendonca BB, Bloise W, Castro M, Tsigos C, Chrousos GP. Brief report: testicular and ovarian resistance to luteinizing hormone caused by inactivating mutations of the luteinizing hormone-receptor gene. N Engl J Med. 1996;334(8):507-512.
- Segaloff DL. Diseases associated with mutations of the human lutropin receptor. Prog Mol Biol Transl Sci. 2009;89:97-114.
- Kossack N, Simoni M, Richter-Unruh A, Themmen AP, Gromoll J. Mutations in a novel, cryptic exon of the luteinizing hormone/chorionic gonadotropin receptor gene cause male pseudohermaphroditism. PLoS Med. 2008;5(4):e88.
- Arnhold IJ, Latronico AC, Batista MC, Izzo CR, Mendonca BB. Clinical features of women with resistance to luteinizing hormone. Clin Endocrinol (Oxf). 1999;51(6):701-707.
- Bruysters M, Christin-Maitre S, Verhoef-Post M, Sultan C, Auger J, Faugeron I, Larue L, Lumbroso S, Themmen AP, Bouchard P. A new LH receptor splice mutation responsible for male hypogonadism with subnormal sperm production in the propositus, and infertility with regular cycles in an affected sister. Hum Reprod. 2008;23(8):1917-1923.
- Miller WL. MECHANISMS IN ENDOCRINOLOGY: Rare defects in adrenal steroidogenesis. Eur J Endocrinol. 2018;179(3):R125-R141.
- Prader A, Gurtner HP. [The syndrome of male pseudohermaphrodism in congenital adrenocortical hyperplasia without overproduction of androgens (adrenal male pseudohermaphrodism)]. Helv Paediatr Acta. 1955;10(4):397-412.
- Miller WL. Molecular biology of steroid hormone synthesis. Endocr Rev. 1988;9(3):295-318.
- Hauffa BP, Miller WL, Grumbach MM, Conte FA, Kaplan SL. Congenital adrenal hyperplasia due to deficient cholesterol side-chain cleavage activity (20, 22-desmolase) in a patient treated for 18 years. Clin Endocrinol (Oxf). 1985;23(5):481-493.
- Bose HS, Sugawara T, Strauss JF, 3rd, Miller WL, International Congenital Lipoid Adrenal Hyperplasia C. The pathophysiology and genetics of congenital lipoid adrenal hyperplasia. N Engl J Med.1996;335(25):1870-1878.
- Abdulhadi-Atwan M, Jean A, Chung WK, Meir K, Ben Neriah Z, Stratigopoulos G, Oberfield SE, Fennoy I, Hirsch HJ, Bhangoo A, Ten S, Lerer I, Zangen DH. Role of a founder c.201_202delCT mutation and new phenotypic features of congenital lipoid adrenal hyperplasia in Palestinians. J Clin Endocrinol Metab. 2007;92(10):4000-4008.
- Fujieda K, Tajima T, Nakae J, Sageshima S, Tachibana K, Suwa S, Sugawara T, Strauss JF, 3rd. Spontaneous puberty in 46,XX subjects with congenital lipoid adrenal hyperplasia. Ovarian steroidogenesis is spared to some extent despite inactivating mutations in the steroidogenic acute regulatory protein (StAR) gene. J Clin Invest. 1997;99(6):1265-1271.
- Hasegawa T, Zhao L, Caron KM, Majdic G, Suzuki T, Shizawa S, Sasano H, Parker KL. Developmental roles of the steroidogenic acute regulatory protein (StAR) as revealed by StAR knockout mice. Mol Endocrinol. 2000;14(9):1462-1471.
- Sugawara T, Lin D, Holt JA, Martin KO, Javitt NB, Miller WL, Strauss JF, 3rd. Structure of the human steroidogenic acute regulatory protein (StAR) gene: StAR stimulates mitochondrial cholesterol 27-hydroxylase activity. Biochemistry. 1995;34(39):12506-12512.
- Miller WL. Congenital lipoid adrenal hyperplasia: the human gene knockout for the steroidogenic acute regulatory protein. J Mol Endocrinol. 1997;19(3):227-240.
- Baker BY, Yaworsky DC, Miller WL. A pH-dependent molten globule transition is required for activity of the steroidogenic acute regulatory protein, StAR. J Biol Chem. 2005;280(50):41753-41760.
- Baker BY, Epand RF, Epand RM, Miller WL. Cholesterol binding does not predict activity of the steroidogenic acute regulatory protein, StAR. J Biol Chem. 2007;282(14):10223-10232.
- Lin D, Sugawara T, Strauss JF, 3rd, Clark BJ, Stocco DM, Saenger P, Rogol A, Miller WL. Role of steroidogenic acute regulatory protein in adrenal and gonadal steroidogenesis. Science.1995;267(5205):1828-1831.
- Baker BY, Lin L, Kim CJ, Raza J, Smith CP, Miller WL, Achermann JC. Nonclassic congenital lipoid adrenal hyperplasia: a new disorder of the steroidogenic acute regulatory protein with very late presentation and normal male genitalia. J Clin Endocrinol Metab. 2006;91(12):4781-4785.
- Nakae J, Tajima T, Sugawara T, Arakane F, Hanaki K, Hotsubo T, Igarashi N, Igarashi Y, Ishii T, Koda N, Kondo T, Kohno H, Nakagawa Y, Tachibana K, Takeshima Y, Tsubouchi K, Strauss JF, 3rd, Fujieda K. Analysis of the steroidogenic acute regulatory protein (StAR) gene in Japanese patients with congenital lipoid adrenal hyperplasia. Hum Mol Genet. 1997;6(4):571-576.
- Fluck CE, Pandey AV, Dick B, Camats N, Fernandez-Cancio M, Clemente M, Gussinye M, Carrascosa A, Mullis PE, Audi L. Characterization of novel StAR (steroidogenic acute regulatory protein) mutations causing non-classic lipoid adrenal hyperplasia. PLoS One. 2011;6(5):e20178.
- Sahakitrungruang T, Soccio RE, Lang-Muritano M, Walker JM, Achermann JC, Miller WL. Clinical, genetic, and functional characterization of four patients carrying partial loss-of-function mutations in the steroidogenic acute regulatory protein (StAR). J Clin Endocrinol Metab. 2010;95(7):3352-3359.
- Metherell LA, Naville D, Halaby G, Begeot M, Huebner A, Nurnberg G, Nurnberg P, Green J, Tomlinson JW, Krone NP, Lin L, Racine M, Berney DM, Achermann JC, Arlt W, Clark AJ. Nonclassic lipoid congenital adrenal hyperplasia masquerading as familial glucocorticoid deficiency. J Clin Endocrinol Metab. 2009;94(10):3865-3871.
- Ishii T, Hori N, Amano N, Aya M, Shibata H, Katsumata N, Hasegawa T. Pubertal and Adult Testicular Functions in Nonclassic Lipoid Congenital Adrenal Hyperplasia: A Case Series and Review. J Endocr Soc. 2019;3(7):1367-1374.
- Morohashi K, Fujii-Kuriyama Y, Okada Y, Sogawa K, Hirose T, Inayama S, Omura T. Molecular cloning and nucleotide sequence of cDNA for mRNA of mitochondrial cytochrome P-450(SCC) of bovine adrenal cortex. Proc Natl Acad Sci U S A. 1984;81(15):4647-4651.
- Hiort O, Holterhus PM, Werner R, Marschke C, Hoppe U, Partsch CJ, Riepe FG, Achermann JC, Struve D. Homozygous disruption of P450 side-chain cleavage (CYP11A1) is associated with prematurity, complete 46,XY sex reversal, and severe adrenal failure. J Clin Endocrinol Metab. 2005;90(1):538-541.
- Tajima T, Fujieda K, Kouda N, Nakae J, Miller WL. Heterozygous mutation in the cholesterol side chain cleavage enzyme (p450scc) gene in a patient with 46,XY sex reversal and adrenal insufficiency. J Clin Endocrinol Metab. 2001;86(8):3820-3825.
- Katsumata N, Ohtake M, Hojo T, Ogawa E, Hara T, Sato N, Tanaka T. Compound heterozygous mutations in the cholesterol side-chain cleavage enzyme gene (CYP11A) cause congenital adrenal insufficiency in humans. J Clin Endocrinol Metab. 2002;87(8):3808-3813.
- Kim CJ, Lin L, Huang N, Quigley CA, AvRuskin TW, Achermann JC, Miller WL. Severe combined adrenal and gonadal deficiency caused by novel mutations in the cholesterol side chain cleavage enzyme, P450scc. J Clin Endocrinol Metab. 2008;93(3):696-702.
- Rubtsov P, Karmanov M, Sverdlova P, Spirin P, Tiulpakov A. A novel homozygous mutation in CYP11A1 gene is associated with late-onset adrenal insufficiency and hypospadias in a 46,XY patient. J Clin Endocrinol Metab. 2009;94(3):936-939.
- Sahakitrungruang T, Tee MK, Blackett PR, Miller WL. Partial defect in the cholesterol side-chain cleavage enzyme P450scc (CYP11A1) resembling nonclassic congenital lipoid adrenal hyperplasia. J Clin Endocrinol Metab. 2011;96(3):792-798.
- Mason JI, Ushijima K, Doody KM, Nagai K, Naville D, Head JR, Milewich L, Rainey WE, Ralph MM. Regulation of expression of the 3 beta-hydroxysteroid dehydrogenases of human placenta and fetal adrenal. J Steroid Biochem Mol Biol. 1993;47(1-6):151-159.
- Bongiovanni AM. The adrenogenital syndrome with deficiency of 3 beta-hydroxysteroid dehydrogenase. J Clin Invest. 1962;41:2086-2092.
- Kalfa N, Gaspari L, Ollivier M, Philibert P, Bergougnoux A, Paris F, Sultan C. Molecular genetics of hypospadias and cryptorchidism recent developments. Clin Genet. 2019;95(1):122-131.
- Guran T, Kara C, Yildiz M, Bitkin EC, Haklar G, Lin JC, Keskin M, Barnard L, Anik A, Catli G, Guven A, Kirel B, Tutunculer F, Onal H, Turan S, Akcay T, Atay Z, Yilmaz GC, Mamadova J, Akbarzade A, Sirikci O, Storbeck KH, Baris T, Chung BC, Bereket A. Revisiting Classical 3β-hydroxysteroid Dehydrogenase 2 Deficiency: Lessons from 31 Pediatric Cases. J Clin Endocrinol Metab. 2020;105(3).
- Mendonça BB, Russell AJ, Vasconcelos-Leite M, Arnhold IJ, Bloise W, Wajchenberg BL, Nicolau W, Sutcliffe RG, Wallace AM. Mutation in 3 beta-hydroxysteroid dehydrogenase type II associated with pseudohermaphroditism in males and premature pubarche or cryptic expression in females. J Mol Endocrinol. 1994;12(1):119-122.
- Sutcliffe RG, Russell AJ, Edwards CR, Wallace AM. Human 3 beta-hydroxysteroid dehydrogenase: genes and phenotypes. J Mol Endocrinol. 1996;17(1):1-5.
- Simard J, Ricketts ML, Gingras S, Soucy P, Feltus FA, Melner MH. Molecular biology of the 3beta-hydroxysteroid dehydrogenase/delta5-delta4 isomerase gene family. Endocr Rev. 2005;26(4):525-582.
- Russell AJ, Wallace AM, Forest MG, Donaldson MD, Edwards CR, Sutcliffe RG. Mutation in the human gene for 3 beta-hydroxysteroid dehydrogenase type II leading to male pseudohermaphroditism without salt loss. J Mol Endocrinol. 1994;12(2):225-237.
- Mendonca BB, Bloise W, Arnhold IJ, Batista MC, Toledo SP, Drummond MC, Nicolau W, Mattar E. Male pseudohermaphroditism due to nonsalt-losing 3 beta-hydroxysteroid dehydrogenase deficiency: gender role change and absence of gynecomastia at puberty. J Steroid Biochem. 1987;28(6):669-675.
- Ladjouze A, Donaldson M, Plotton I, Djenane N, Mohammedi K, Tardy-Guidollet V, Mallet D, Boulesnane K, Bouzerar Z, Morel Y, Roucher-Boulez F. Genotype, Mortality, Morbidity, and Outcomes of 3β-Hydroxysteroid Dehydrogenase Deficiency in Algeria. Front Endocrinol (Lausanne).2022;13:867073.
- Miller WL. The syndrome of 17,20 lyase deficiency. J Clin Endocrinol Metab. 2012;97(1):59-67.
- Biglieri EG, Herron MA, Brust N. 17-hydroxylation deficiency in man. J Clin Invest. 1966;45(12):1946-1954.
- New MI. Male pseudohermaphroditism due to 17 alpha-hydroxylase deficiency. J Clin Invest.1970;49(10):1930-1941.
- Auchus RJ. The uncommon forms of congenital adrenal hyperplasia. Curr Opin Endocrinol Diabetes Obes. 2022;29(3):263-270.
- Yanase T, Simpson ER, Waterman MR. 17 alpha-hydroxylase/17,20-lyase deficiency: from clinical investigation to molecular definition. Endocr Rev. 1991;12(1):91-108.
- Auchus RJ. The genetics, pathophysiology, and management of human deficiencies of P450c17. Endocrinol Metab Clin North Am. 2001;30(1):101-119, vii.
- Zachmann M. Recent aspects of steroid biosynthesis in male sex differentiation. Clinical studies. Horm Res. 1992;38(5-6):211-216.
- Martin RM, Lin CJ, Costa EM, de Oliveira ML, Carrilho A, Villar H, Longui CA, Mendonca BB. P450c17 deficiency in Brazilian patients: biochemical diagnosis through progesterone levels confirmed by CYP17 genotyping. J Clin Endocrinol Metab. 2003;88(12):5739-5746.
- Matteson KJ, Picado-Leonard J, Chung BC, Mohandas TK, Miller WL. Assignment of the gene for adrenal P450c17 (steroid 17 alpha-hydroxylase/17,20 lyase) to human chromosome 10. J Clin Endocrinol Metab. 1986;63(3):789-791.
- Rosa S, Duff C, Meyer M, Lang-Muritano M, Balercia G, Boscaro M, Topaloglu AK, Mioni R, Fallo F, Zuliani L, Mantero F, Schoenle EJ, Biason-Lauber A. P450c17 deficiency: clinical and molecular characterization of six patients. J Clin Endocrinol Metab. 2007;92(3):1000-1007.
- Peterson RE, Imperato-McGinley J, Gautier T, Shackleton C. Male pseudohermaphroditism due to multiple defects in steroid-biosynthetic microsomal mixed-function oxidases. A new variant of congenital adrenal hyperplasia. N Engl J Med. 1985;313(19):1182-1191.
- Arlt W, Walker EA, Draper N, Ivison HE, Ride JP, Hammer F, Chalder SM, Borucka-Mankiewicz M, Hauffa BP, Malunowicz EM, Stewart PM, Shackleton CH. Congenital adrenal hyperplasia caused by mutant P450 oxidoreductase and human androgen synthesis: analytical study. Lancet.2004;363(9427):2128-2135.
- Shephard EA, Phillips IR, Santisteban I, West LF, Palmer CN, Ashworth A, Povey S. Isolation of a human cytochrome P-450 reductase cDNA clone and localization of the corresponding gene to chromosome 7q11.2. Ann Hum Genet. 1989;53(4):291-301.
- Reardon W, Smith A, Honour JW, Hindmarsh P, Das D, Rumsby G, Nelson I, Malcolm S, Ades L, Sillence D, Kumar D, DeLozier-Blanchet C, McKee S, Kelly T, McKeehan WL, Baraitser M, Winter RM. Evidence for digenic inheritance in some cases of Antley-Bixler syndrome? J Med Genet.2000;37(1):26-32.
- Huang N, Pandey AV, Agrawal V, Reardon W, Lapunzina PD, Mowat D, Jabs EW, Van Vliet G, Sack J, Fluck CE, Miller WL. Diversity and function of mutations in p450 oxidoreductase in patients with Antley-Bixler syndrome and disordered steroidogenesis. Am J Hum Genet. 2005;76(5):729-749.
- Schmidt K, Hughes C, Chudek JA, Goodyear SR, Aspden RM, Talbot R, Gundersen TE, Blomhoff R, Henderson C, Wolf CR, Tickle C. Cholesterol metabolism: the main pathway acting downstream of cytochrome P450 oxidoreductase in skeletal development of the limb. Mol Cell Biol. 2009;29(10):2716-2729.
- Finkielstain GP, Vieites A, Bergadá I, Rey RA. Disorders of Sex Development of Adrenal Origin. Front Endocrinol (Lausanne). 2021;12:770782.
- Idkowiak J, O'Riordan S, Reisch N, Malunowicz EM, Collins F, Kerstens MN, Kohler B, Graul-Neumann LM, Szarras-Czapnik M, Dattani M, Silink M, Shackleton CH, Maiter D, Krone N, Arlt W. Pubertal presentation in seven patients with congenital adrenal hyperplasia due to P450 oxidoreductase deficiency. J Clin Endocrinol Metab. 2011;96(3):E453-462.
- Zachmann M, Vollmin JA, Hamilton W, Prader A. Steroid 17,20-desmolase deficiency: a new cause of male pseudohermaphroditism. Clin Endocrinol (Oxf). 1972;1(4):369-385.
- Auchus RJ. Steroid 17-hydroxylase and 17,20-lyase deficiencies, genetic and pharmacologic. J Steroid Biochem Mol Biol. 2017;165(Pt A):71-78.
- Geller DH, Auchus RJ, Miller WL. P450c17 mutations R347H and R358Q selectively disrupt 17,20-lyase activity by disrupting interactions with P450 oxidoreductase and cytochrome b5. Mol Endocrinol.1999;13(1):167-175.
- Hegesh E, Hegesh J, Kaftory A. Congenital methemoglobinemia with a deficiency of cytochrome b5. N Engl J Med. 1986;314(12):757-761.
- Idkowiak J, Randell T, Dhir V, Patel P, Shackleton CH, Taylor NF, Krone N, Arlt W. A missense mutation in the human cytochrome b5 gene causes 46,XY disorder of sex development due to true isolated 17,20 lyase deficiency. J Clin Endocrinol Metab. 2012;97(3):E465-475.
- Saez JM, De Peretti E, Morera AM, David M, Bertrand J. Familial male pseudohermaphroditism with gynecomastia due to a testicular 17-ketosteroid reductase defect. I. Studies in vivo. J Clin Endocrinol Metab. 1971;32(5):604-610.
- Boehmer AL, Brinkmann AO, Sandkuijl LA, Halley DJ, Niermeijer MF, Andersson S, de Jong FH, Kayserili H, de Vroede MA, Otten BJ, Rouwé CW, Mendonça BB, Rodrigues C, Bode HH, de Ruiter PE, Delemarre-van de Waal HA, Drop SL. 17Beta-hydroxysteroid dehydrogenase-3 deficiency: diagnosis, phenotypic variability, population genetics, and worldwide distribution of ancient and de novo mutations. J Clin Endocrinol Metab. 1999;84(12):4713-4721.
- George MM, New MI, Ten S, Sultan C, Bhangoo A. The clinical and molecular heterogeneity of 17betaHSD-3 enzyme deficiency. Horm Res Paediatr. 2010;74(4):229-240.
- Andersson S, Moghrabi N. Physiology and molecular genetics of 17 beta-hydroxysteroid dehydrogenases. Steroids. 1997;62(1):143-147.
- Andersson S, Geissler WM, Wu L, Davis DL, Grumbach MM, New MI, Schwarz HP, Blethen SL, Mendonca BB, Bloise W, Witchel SF, Cutler GB, Griffin JE, Wilson JD, Russel DW. Molecular genetics and pathophysiology of 17 beta-hydroxysteroid dehydrogenase 3 deficiency. J Clin Endocrinol Metab.1996;81(1):130-136.
- Lee YS, Kirk JM, Stanhope RG, Johnston DI, Harland S, Auchus RJ, Andersson S, Hughes IA. Phenotypic variability in 17beta-hydroxysteroid dehydrogenase-3 deficiency and diagnostic pitfalls. Clin Endocrinol (Oxf). 2007;67(1):20-28.
- Mendonca BB, Gomes NL, Costa EM, Inacio M, Martin RM, Nishi MY, Carvalho FM, Tibor FD, Domenice S. 46,XY disorder of sex development (DSD) due to 17β-hydroxysteroid dehydrogenase type 3 deficiency. J Steroid Biochem Mol Biol. 2017;165(Pt A):79-85.
- Mendonca BB, Inacio M, Arnhold IJ, Costa EM, Bloise W, Martin RM, Denes FT, Silva FA, Andersson S, Lindqvist A, Wilson JD. Male pseudohermaphroditism due to 17 beta-hydroxysteroid dehydrogenase 3 deficiency. Diagnosis, psychological evaluation, and management. Medicine (Baltimore).2000;79(5):299-309.
- Bertelloni S, Balsamo A, Giordani L, Fischetto R, Russo G, Delvecchio M, Gennari M, Nicoletti A, Maggio MC, Concolino D, Cavallo L, Cicognani A, Chiumello G, Hiort O, Baroncelli GI, Faienza MF. 17beta-Hydroxysteroid dehydrogenase-3 deficiency: from pregnancy to adolescence. J Endocrinol Invest. 2009;32(8):666-670.
- Khattab A, Yuen T, Yau M, Domenice S, Frade Costa EM, Diya K, Muhuri D, Pina CE, Nishi MY, Yang AC, de Mendonça BB, New MI. Pitfalls in hormonal diagnosis of 17-beta hydroxysteroid dehydrogenase III deficiency. J Pediatr Endocrinol Metab. 2015;28(5-6):623-628.
- McKeever BM, Hawkins BK, Geissler WM, Wu L, Sheridan RP, Mosley RT, Andersson S. Amino acid substitution of arginine 80 in 17beta-hydroxysteroid dehydrogenase type 3 and its effect on NADPH cofactor binding and oxidation/reduction kinetics. Biochim Biophys Acta. 2002;1601(1):29-37.
- Cocchetti C, Baldinotti F, Romani A, Ristori J, Mazzoli F, Vignozzi L, Maggi M, Fisher AD. A Novel Compound Heterozygous Mutation of HSD17B3 Gene Identified in a Patient With 46,XY Difference of Sexual Development. Sex Med. 2022;10(4):100522.
- von Spreckelsen B, Aksglaede L, Johannsen TH, Nielsen JE, Main KM, Jørgensen A, Jensen RB. Prepubertal and pubertal gonadal morphology, expression of cell lineage markers and hormonal evaluation in two 46,XY siblings with 17β-hydroxysteroid dehydrogenase 3 deficiency. J Pediatr Endocrinol Metab. 2022;35(7):953-961.
- Jahagirdar R, Khadilkar V, Deshpande R, Lohiya N. Clinical, Etiological and Laboratory Profile of Children with Disorders of Sexual Development (DSD)-Experience from a Tertiary Pediatric Endocrine Unit in Western India. Indian J Endocrinol Metab. 2021;25(1):48-53.
- Manyas H, Eroğlu Filibeli B, Ayrancı İ, Güvenç MS, Dündar BN, Çatlı G. Early and late diagnoses of 17β-Hydroxysteroid dehydrogenase type-3 deficiency in two unrelated patients. Andrologia.2021;53(6):e14017.
- Rösler A, Kohn G. Male pseudohermaphroditism due to 17 beta-hydroxysteroid dehydrogenase deficiency: studies on the natural history of the defect and effect of androgens on gender role. J Steroid Biochem. 1983;19(1B):663-674.
- Cools M, Drop SL, Wolffenbuttel KP, Oosterhuis JW, Looijenga LH. Germ cell tumors in the intersex gonad: old paths, new directions, moving frontiers. Endocr Rev. 2006;27(5):468-484.
- Looijenga LH, Hersmus R, Oosterhuis JW, Cools M, Drop SL, Wolffenbuttel KP. Tumor risk in disorders of sex development (DSD). Best Pract Res Clin Endocrinol Metab. 2007;21(3):480-495.
- Kathrins M, Kolon TF. Malignancy in disorders of sex development. Transl Androl Urol. 2016;5(5):794-798.
- Abacı A, Çatlı G, Berberoğlu M. Gonadal malignancy risk and prophylactic gonadectomy in disorders of sexual development. J Pediatr Endocrinol Metab. 2015;28(9-10):1019-1027.
- Auchus RJ. The backdoor pathway to dihydrotestosterone. Trends Endocrinol Metab. 2004;15(9):432-438.
- Wilson JD, Auchus RJ, Leihy MW, Guryev OL, Estabrook RW, Osborn SM, Shaw G, Renfree MB. 5alpha-androstane-3alpha,17beta-diol is formed in tammar wallaby pouch young testes by a pathway involving 5alpha-pregnane-3alpha,17alpha-diol-20-one as a key intermediate. Endocrinology.2003;144(2):575-580.
- Lee HG, Kim CJ. Classic and backdoor pathways of androgen biosynthesis in human sexual development. Ann Pediatr Endocrinol Metab. 2022;27(2):83-89.
- Biswas MG, Russell DW. Expression cloning and characterization of oxidative 17beta- and 3alpha-hydroxysteroid dehydrogenases from rat and human prostate. J Biol Chem. 1997;272(25):15959-15966.
- Dufort I, Soucy P, Labrie F, Luu-The V. Molecular cloning of human type 3 3 alpha-hydroxysteroid dehydrogenase that differs from 20 alpha-hydroxysteroid dehydrogenase by seven amino acids. Biochem Biophys Res Commun. 1996;228(2):474-479.
- Fluck CE, Meyer-Boni M, Pandey AV, Kempna P, Miller WL, Schoenle EJ, Biason-Lauber A. Why boys will be boys: two pathways of fetal testicular androgen biosynthesis are needed for male sexual differentiation. Am J Hum Genet. 2011;89(2):201-218.
- Mares L, Vilchis F, Chavez B, Ramos L. Molecular genetic analysis of AKR1C2-4 and HSD17B6 genes in subjects 46,XY with hypospadias. J Pediatr Urol. 2020;16(5):689 e681-689 e612.
- Penning TM. The aldo-keto reductases (AKRs): Overview. Chem Biol Interact. 2015;234:236-246.
- NOWAKOWSKI H, LENZ W. Genetic aspects in male hypogonadism. Recent Prog Horm Res.1961;17:53-95.
- Imperato-McGinley J, Guerrero L, Gautier T, Peterson RE. Steroid 5alpha-reductase deficiency in man: an inherited form of male pseudohermaphroditism. Science. 1974;186(4170):1213-1215.
- Walsh PC, Madden JD, Harrod MJ, Goldstein JL, MacDonald PC, Wilson JD. Familial incomplete male pseudohermaphroditism, type 2. Decreased dihydrotestosterone formation in pseudovaginal perineoscrotal hypospadias. N Engl J Med. 1974;291(18):944-949.
- Andersson S, Russell DW. Structural and biochemical properties of cloned and expressed human and rat steroid 5 alpha-reductases. Proc Natl Acad Sci U S A. 1990;87(10):3640-3644.
- Imperato-McGinley J, Miller M, Wilson JD, Peterson RE, Shackleton C, Gajdusek DC. A cluster of male pseudohermaphrodites with 5 alpha-reductase deficiency in Papua New Guinea. Clin Endocrinol (Oxf).1991;34(4):293-298.
- Thigpen AE, Davis DL, Milatovich A, Mendonca BB, Imperato-McGinley J, Griffin JE, Francke U, Wilson JD, Russell DW. Molecular genetics of steroid 5 alpha-reductase 2 deficiency. J Clin Invest.1992;90(3):799-809.
- Batista RL, Mendonca BB. Integrative and Analytical Review of the 5-Alpha-Reductase Type 2 Deficiency Worldwide. Appl Clin Genet. 2020;13:83-96.
- Batista RL, Mendonca BB. The Molecular Basis of 5alpha-Reductase Type 2 Deficiency. Sex Dev.2022:1-13.
- Mendonca BB, Batista RL, Domenice S, Costa EM, Arnhold IJ, Russell DW, Wilson JD. Steroid 5α-reductase 2 deficiency. J Steroid Biochem Mol Biol. 2016;163:206-211.
- Avendaño A, Paradisi I, Cammarata-Scalisi F, Callea M. 5-α-Reductase type 2 deficiency: is there a genotype-phenotype correlation? A review. Hormones (Athens). 2018;17(2):197-204.
- Gui B, Song Y, Su Z, Luo FH, Chen L, Wang X, Chen R, Yang Y, Wang J, Zhao X, Fan L, Liu X, Wang Y, Chen S, Gong C. New insights into 5α-reductase type 2 deficiency based on a multi-centre study: regional distribution and genotype-phenotype profiling of. J Med Genet. 2019;56(10):685-692.
- Berra M, Williams EL, Muroni B, Creighton SM, Honour JW, Rumsby G, Conway GS. Recognition of 5α-reductase-2 deficiency in an adult female 46XY DSD clinic. Eur J Endocrinol. 2011;164(6):1019-1025.
- Shabir I, Khurana ML, Joseph AA, Eunice M, Mehta M, Ammini AC. Phenotype, genotype and gender identity in a large cohort of patients from India with 5α-reductase 2 deficiency. Andrology.2015;3(6):1132-1139.
- Cheng J, Lin R, Zhang W, Liu G, Sheng H, Li X, Zhou Z, Mao X, Liu L. Phenotype and molecular characteristics in 45 Chinese children with 5α-reductase type 2 deficiency from South China. Clin Endocrinol (Oxf). 2015;83(4):518-526.
- Mendonca BB, Inacio M, Costa EM, Arnhold IJ, Silva FA, Nicolau W, Bloise W, Russel DW, Wilson JD. Male pseudohermaphroditism due to steroid 5alpha-reductase 2 deficiency. Diagnosis, psychological evaluation, and management. Medicine (Baltimore). 1996;75(2):64-76.
- Bertelloni S, Baldinotti F, Russo G, Ghirri P, Dati E, Michelucci A, Moscuzza F, Meroni S, Colombo I, Sessa MR, Baroncelli GI. 5α-Reductase-2 Deficiency: Clinical Findings, Endocrine Pitfalls, and Genetic Features in a Large Italian Cohort. Sex Dev. 2016;10(1):28-36.
- Vilchis F, Méndez JP, Canto P, Lieberman E, Chávez B. Identification of missense mutations in the SRD5A2 gene from patients with steroid 5alpha-reductase 2 deficiency. Clin Endocrinol (Oxf).2000;52(3):383-387.
- Imperato-McGinley J. 5alpha-reductase-2 deficiency and complete androgen insensitivity: lessons from nature. Adv Exp Med Biol. 2002;511:121-131; discussion 131-124.
- Walter KN, Kienzle FB, Frankenschmidt A, Hiort O, Wudy SA, van der Werf-Grohmann N, Superti-Furga A, Schwab KO. Difficulties in diagnosis and treatment of 5alpha-reductase type 2 deficiency in a newborn with 46,XY DSD. Horm Res Paediatr. 2010;74(1):67-71.
- Hochberg Z, Chayen R, Reiss N, Falik Z, Makler A, Munichor M, Farkas A, Goldfarb H, Ohana N, Hiort O. Clinical, biochemical, and genetic findings in a large pedigree of male and female patients with 5 alpha-reductase 2 deficiency. J Clin Endocrinol Metab. 1996;81(8):2821-2827.
- Costa EM, Domenice S, Sircili MH, Inacio M, Mendonca BB. DSD due to 5α-reductase 2 deficiency - from diagnosis to long term outcome. Semin Reprod Med. 2012;30(5):427-431.
- Mendonca BB, Batista RL, Domenice S, Costa EM, Arnhold IJ, Russell DW, Wilson JD. Reprint of "Steroid 5α-reductase 2 deficiency". J Steroid Biochem Mol Biol. 2017;165(Pt A):95-100.
- Gomes NL, Batista RL, Nishi MY, Lerario AM, Silva TE, Narcizo AM, Benedetti AFF, Funari MFA, Junior JAF, Moraes DR, Quintao LML, Montenegro LR, Ferrari MTM, Jorge AA, Arnhold IJP, Costa EMF, Domenice S, Mendonca BB. Contribution of clinical and genetic approaches for diagnosing 209 index cases with 46,XY Differences of Sex Development. J Clin Endocrinol Metab. 2022.
- Chan AO, But BW, Lee CY, Lam YY, Ng KL, Tung JY, Kwan EY, Chan YK, Tsui TK, Lam AL, Tse WY, Cheung PT, Shek CC. Diagnosis of 5α-reductase 2 deficiency: is measurement of dihydrotestosterone essential? Clin Chem. 2013;59(5):798-806.
- Cohen-Kettenis PT. Psychosocial and psychosexual aspects of disorders of sex development. Best Pract Res Clin Endocrinol Metab. 2010;24(2):325-334.
- Ahmed SF, Achermann JC, Arlt W, Balen A, Conway G, Edwards Z, Elford S, Hughes IA, Izatt L, Krone N, Miles H, O'Toole S, Perry L, Sanders C, Simmonds M, Watt A, Willis D. Society for Endocrinology UK guidance on the initial evaluation of an infant or an adolescent with a suspected disorder of sex development (Revised 2015). Clin Endocrinol (Oxf). 2016;84(5):771-788.
- Costa EM, Mendonca BB, Inácio M, Arnhold IJ, Silva FA, Lodovici O. Management of ambiguous genitalia in pseudohermaphrodites: new perspectives on vaginal dilation. Fertil Steril. 1997;67(2):229-232.
- Sircili MH, e Silva FA, Costa EM, Brito VN, Arnhold IJ, Dénes FT, Inacio M, de Mendonca BB. Long-term surgical outcome of masculinizing genitoplasty in large cohort of patients with disorders of sex development. J Urol. 2010;184(3):1122-1127.
- Cohen-Kettenis PT. Gender change in 46,XY persons with 5alpha-reductase-2 deficiency and 17beta-hydroxysteroid dehydrogenase-3 deficiency. Arch Sex Behav. 2005;34(4):399-410.
- Loch Batista R, Inácio M, Prado Arnhold IJ, Gomes NL, Diniz Faria JA, Rodrigues de Moraes D, Frade Costa EM, Domenice S, Bilharinho Mendonça B. Psychosexual Aspects, Effects of Prenatal Androgen Exposure, and Gender Change in 46,XY Disorders of Sex Development. J Clin Endocrinol Metab.2019;104(4):1160-1170.
- Meyer-Bahlburg HF, Baratz Dalke K, Berenbaum SA, Cohen-Kettenis PT, Hines M, Schober JM. Gender Assignment, Reassignment and Outcome in Disorders of Sex Development: Update of the 2005 Consensus Conference. Horm Res Paediatr. 2016;85(2):112-118.
- Fisher AD, Ristori J, Fanni E, Castellini G, Forti G, Maggi M. Gender identity, gender assignment and reassignment in individuals with disorders of sex development: a major of dilemma. J Endocrinol Invest.2016;39(11):1207-1224.
- Mendonca BB. Gender assignment in patients with disorder of sex development. Curr Opin Endocrinol Diabetes Obes. 2014;21(6):511-514.
- Amaral RC, Inacio M, Brito VN, Bachega TA, Domenice S, Arnhold IJ, Madureira G, Gomes L, Costa EM, Mendonca BB. Quality of life of patients with 46,XX and 46,XY disorders of sex development. Clin Endocrinol (Oxf). 2015;82(2):159-164.
- Cassia Amaral R, Inacio M, Brito VN, Bachega TA, Oliveira AA, Domenice S, Denes FT, Sircili MH, Arnhold IJ, Madureira G, Gomes L, Costa EM, Mendonca BB. Quality of life in a large cohort of adult Brazilian patients with 46,XX and 46,XY disorders of sex development from a single tertiary centre. Clin Endocrinol (Oxf). 2015;82(2):274-279.
- Batista RL, Costa EMF, Rodrigues AS, Gomes NL, Faria JA, Nishi MY, Arnhold IJP, Domenice S, Mendonca BB. Androgen insensitivity syndrome: a review. Arch Endocrinol Metab. 2018;62(2):227-235.
- Hiort O. Clinical and molecular aspects of androgen insensitivity. Endocr Dev. 2013;24:33-40.
- Mongan NP, Tadokoro-Cuccaro R, Bunch T, Hughes IA. Androgen insensitivity syndrome. Best Pract Res Clin Endocrinol Metab. 2015;29(4):569-580.
- Hughes IA, Werner R, Bunch T, Hiort O. Androgen insensitivity syndrome. Semin Reprod Med.2012;30(5):432-442.
- Batista RL, Craveiro FL, Ramos RM, Mendonca BB. Mild Androgen Insensitivity Syndrome: The Current Landscape. Endocr Pract. 2022.
- Yu X, Yi P, Hamilton RA, Shen H, Chen M, Foulds CE, Mancini MA, Ludtke SJ, Wang Z, O'Malley BW. Structural Insights of Transcriptionally Active, Full-Length Androgen Receptor Coactivator Complexes. Mol Cell. 2020;79(5):812-823.e814.
- Clinckemalie L, Vanderschueren D, Boonen S, Claessens F. The hinge region in androgen receptor control. Mol Cell Endocrinol. 2012;358(1):1-8.
- Schlanger S, Heemers HV. Functional Studies on Steroid Receptors. Methods Mol Biol. 2018;1786:117-130.
- Tan MH, Li J, Xu HE, Melcher K, Yong EL. Androgen receptor: structure, role in prostate cancer and drug discovery. Acta Pharmacol Sin. 2015;36(1):3-23.
- Chmelar R, Buchanan G, Need EF, Tilley W, Greenberg NM. Androgen receptor coregulators and their involvement in the development and progression of prostate cancer. Int J Cancer. 2007;120(4):719-733.
- Heinlein CA, Chang C. Androgen receptor (AR) coregulators: an overview. Endocr Rev.2002;23(2):175-200.
- Tirabassi G, Cignarelli A, Perrini S, Delli Muti N, Furlani G, Gallo M, Pallotti F, Paoli D, Giorgino F, Lombardo F, Gandini L, Lenzi A, Balercia G. Influence of CAG Repeat Polymorphism on the Targets of Testosterone Action. Int J Endocrinol. 2015;2015:298107.
- Huang G, Shan W, Zeng L, Huang L. Androgen receptor gene CAG repeat polymorphism and risk of isolated hypospadias: results from a meta-analysis. Genet Mol Res. 2015;14(1):1580-1588.
- Malek EG, Salameh JS, Makki A. Kennedy's disease: an under-recognized motor neuron disorder. Acta Neurol Belg. 2020;120(6):1289-1295.
- Paz-Y-Miño C, Robles P, Salazar C, Leone PE, García-Cárdenas JM, Naranjo M, López-Cortés A. Positive association of the androgen receptor CAG repeat length polymorphism with the risk of prostate cancer. Mol Med Rep. 2016;14(2):1791-1798.
- Gottlieb B, Beitel LK, Nadarajah A, Paliouras M, Trifiro M. The androgen receptor gene mutations database: 2012 update. Hum Mutat. 2012;33(5):887-894.
- Hornig NC, Holterhus PM. Molecular basis of androgen insensitivity syndromes. Mol Cell Endocrinol.2021;523:111146.
- Batista RL, Yamaguchi K, di Santi Rodrigues A, Nishi MY, Goodier JL, Carvalho LR, Domenice S, Costa EMF, Hazazian H, Mendonca BB. Mobile DNA in Endocrinology: LINE-1 retrotransposon causing Partial Androgen Insensitivity Syndrome. J Clin Endocrinol Metab. 2019.
- Hornig NC, de Beaufort C, Denzer F, Cools M, Wabitsch M, Ukat M, Kulle AE, Schweikert HU, Werner R, Hiort O, Audi L, Siebert R, Ammerpohl O, Holterhus PM. A Recurrent Germline Mutation in the 5'UTR of the Androgen Receptor Causes Complete Androgen Insensitivity by Activating Aberrant uORF Translation. PLoS One. 2016;11(4):e0154158.
- Batista RL, di Santi Rodrigues A, Nishi MY, Gomes NLRA, Faria JAD, de Moraes DR, Carvalho LR, Frade EMC, Domenice S, de Mendonca BB. A Recurrent Synonymous Mutation in the Human Androgen Receptor Gene Causing Complete Androgen Insensitivity Syndrome. J Steroid Biochem Mol Biol. 2017.
- Känsäkoski J, Jääskeläinen J, Jääskeläinen T, Tommiska J, Saarinen L, Lehtonen R, Hautaniemi S, Frilander MJ, Palvimo JJ, Toppari J, Raivio T. Complete androgen insensitivity syndrome caused by a deep intronic pseudoexon-activating mutation in the androgen receptor gene. Sci Rep. 2016;6:32819.
- Hornig NC, Ukat M, Schweikert HU, Hiort O, Werner R, Drop SL, Cools M, Hughes IA, Audi L, Ahmed SF, Demiri J, Rodens P, Worch L, Wehner G, Kulle AE, Dunstheimer D, Müller-Roßberg E, Reinehr T, Hadidi AT, Eckstein AK, van der Horst C, Seif C, Siebert R, Ammerpohl O, Holterhus PM. Identification of an AR Mutation-Negative Class of Androgen Insensitivity by Determining Endogenous AR Activity. J Clin Endocrinol Metab. 2016;101(11):4468-4477.
- Riskin A, Koren I, Bader D, Grün M, Dar H, Leibovitz Z, Kugelman A, Hiort O. The approach to a neonate with a possible prenatal diagnosis of androgen insensitivity syndrome. J Pediatr Endocrinol Metab. 2006;19(12):1437-1443.
- Batista RL. Complete Androgen Insensitivity in Girls with Inguinal Hernias: A Serendipity Opportunity for Early Diagnosis. J Invest Surg. 2019:1-2.
- Oakes MB, Eyvazzadeh AD, Quint E, Smith YR. Complete androgen insensitivity syndrome--a review. J Pediatr Adolesc Gynecol. 2008;21(6):305-310.
- Döhnert U, Wünsch L, Hiort O. Gonadectomy in Complete Androgen Insensitivity Syndrome: Why and When? Sex Dev. 2017.
- Tack LJW, Maris E, Looijenga LHJ, Hannema SE, Audi L, Köhler B, Holterhus PM, Riedl S, Wisniewski A, Flück CE, Davies JH, T Apos Sjoen G, Lucas-Herald AK, Evliyaoglu O, Krone N, Iotova V, Marginean O, Balsamo A, Verkauskas G, Weintrob N, Ellaithi M, Nordenström A, Verrijn Stuart A, Kluivers KB, Wolffenbuttel KP, Ahmed SF, Cools M. Management of Gonads in Adults with Androgen Insensitivity: An International Survey. Horm Res Paediatr. 2018:1-11.
- Cools M, Looijenga L. Update on the Pathophysiology and Risk Factors for the Development of Malignant Testicular Germ Cell Tumors in Complete Androgen Insensitivity Syndrome. Sex Dev. 2017.
- Deans R, Creighton SM, Liao LM, Conway GS. Timing of gonadectomy in adult women with complete androgen insensitivity syndrome (CAIS): patient preferences and clinical evidence. Clin Endocrinol (Oxf). 2012;76(6):894-898.
- Danilovic DL, Correa PH, Costa EM, Melo KF, Mendonca BB, Arnhold IJ. Height and bone mineral density in androgen insensitivity syndrome with mutations in the androgen receptor gene. Osteoporos Int. 2007;18(3):369-374.
- Lucas-Herald A, Bertelloni S, Juul A, Bryce J, Jiang J, Rodie M, Sinnott R, Boroujerdi M, Lindhardt-Johansen M, Hiort O, Holterhus PM, Cools M, Guaragna-Filho G, Guerra-Junior G, Weintrob N, Hannema S, Drop S, Guran T, Darendeliler F, Nordenstrom A, Hughes IA, Acerini C, Tadokoro-Cuccaro R, Ahmed SF. The Long Term Outcome Of Boys With Partial Androgen Insensitivity Syndrome And A Mutation In The Androgen Receptor Gene. J Clin Endocrinol Metab. 2016:jc20161372.
- Gulía C, Baldassarra S, Zangari A, Briganti V, Gigli S, Gaffi M, Signore F, Vallone C, Nucciotti R, Costantini FM, Pizzuti A, Bernardo S, Porrello A, Piergentili R. Androgen insensitivity syndrome. Eur Rev Med Pharmacol Sci. 2018;22(12):3873-3887.
- Josso N, di Clemente N, Gouedard L. Anti-Mullerian hormone and its receptors. Mol Cell Endocrinol.2001;179(1-2):25-32.
- Rey R. Anti-Müllerian hormone in disorders of sex determination and differentiation. Arq Bras Endocrinol Metabol. 2005;49(1):26-36.
- Loeff DS, Imbeaud S, Reyes HM, Meller JL, Rosenthal IM. Surgical and genetic aspects of persistent mullerian duct syndrome. J Pediatr Surg. 1994;29(1):61-65.
- Josso N, di Clemente N. TGF-beta Family Members and Gonadal Development. Trends Endocrinol Metab. 1999;10(6):216-222.
- Imbeaud S, Carre-Eusebe D, Rey R, Belville C, Josso N, Picard JY. Molecular genetics of the persistent mullerian duct syndrome: a study of 19 families. Hum Mol Genet. 1994;3(1):125-131.
- Imbeaud S, Faure E, Lamarre I, Mattei MG, di Clemente N, Tizard R, Carre-Eusebe D, Belville C, Tragethon L, Tonkin C, Nelson J, McAuliffe M, Bidart JM, Lababidi A, Josso N, Cate RL, Picard JY. Insensitivity to anti-mullerian hormone due to a mutation in the human anti-mullerian hormone receptor. Nat Genet. 1995;11(4):382-388.
- Orós-Millán ME, Muñoz-Calvo MT, Nishi MY, Bilharinho Mendonca B, Argente J. [Persistent Müllerian duct syndrome due to a mutation in the anti-Müllerian hormone receptor gene (AMHR2)]. An Pediatr (Barc). 2016.
- Saleem M, Ather U, Mirza B, Iqbal S, Sheikh A, Shaukat M, Sheikh MT, Ahmad F, Rehan T. Persistent mullerian duct syndrome: A 24-year experience. J Pediatr Surg. 2016;51(10):1721-1724.
- Aarskog D. Maternal progestins as a possible cause of hypospadias. N Engl J Med. 1979;300(2):75-78.
- Driscoll SG, Taylor SH. Effects of prenatal maternal estrogen on the male urogenital system. Obstet Gynecol. 1980;56(5):537-542.
- Watanabe M, Yoshida R, Ueoka K, Aoki K, Sasagawa I, Hasegawa T, Sueoka K, Kamatani N, Yoshimura Y, Ogata T. Haplotype analysis of the estrogen receptor 1 gene in male genital and reproductive abnormalities. Hum Reprod. 2007;22(5):1279-1284.
- Rider CV, Furr J, Wilson VS, Gray LE, Jr. A mixture of seven antiandrogens induces reproductive malformations in rats. Int J Androl. 2008;31(2):249-262.
- Vilela ML, Willingham E, Buckley J, Liu BC, Agras K, Shiroyanagi Y, Baskin LS. Endocrine disruptors and hypospadias: role of genistein and the fungicide vinclozolin. Urology. 2007;70(3):618-621.
- Fredell L, Lichtenstein P, Pedersen NL, Svensson J, Nordenskjold A. Hypospadias is related to birth weight in discordant monozygotic twins. J Urol. 1998;160(6 Pt 1):2197-2199.
- Francois I, van Helvoirt M, de Zegher F. Male pseudohermaphroditism related to complications at conception, in early pregnancy or in prenatal growth. Horm Res. 1999;51(2):91-95.
- Mendonca BB, Billerbeck AE, de Zegher F. Nongenetic male pseudohermaphroditism and reduced prenatal growth. N Engl J Med. 2001;345(15):1135.
- Rossignol S, Netchine I, Le Bouc Y, Gicquel C. Epigenetics in Silver-Russell syndrome. Best Pract Res Clin Endocrinol Metab. 2008;22(3):403-414.
- Morel Y, Rey R, Teinturier C, Nicolino M, Michel-Calemard L, Mowszowicz I, Jaubert F, Fellous M, Chaussain JL, Chatelain P, David M, Nihoul-Fekete C, Forest MG, Josso N. Aetiological diagnosis of male sex ambiguity: a collaborative study. Eur J Pediatr. 2002;161(1):49-59.
- Main KM, Jensen RB, Asklund C, Hoi-Hansen CE, Skakkebaek NE. Low birth weight and male reproductive function. Horm Res. 2006;65 Suppl 3:116-122.
- Leitao Braga B, Lisboa Gomes N, Nishi MY, Freire BL, Batista RL, JA DFJ, Funari MFA, Figueredo Benedetti AF, de Moraes Narcizo A, Cavalca Cardoso L, Lerario AM, Guerra-Junior G, Frade Costa EM, Domenice S, Jorge AAL, Mendonca BB. Variants in 46,XY DSD-Related Genes in Syndromic and Non-Syndromic Small for Gestational Age Children with Hypospadias. Sex Dev. 2022;16(1):27-33.
- Scarpa MG, Grazia MD, Tornese G. 46,XY ovotesticular disorders of sex development: A therapeutic challenge. Pediatr Rep. 2017;9(4):7085.
- van der Horst HJ, de Wall LL. Hypospadias, all there is to know. Eur J Pediatr. 2017;176(4):435-441.
- Mole RJ, Nash S, MacKenzie DN. Hypospadias. BMJ. 2020;369:m2070.
- Hutson JM. Cryptorchidism and Hypospadias. In: Feingold KR, Anawalt B, Boyce A, Chrousos G, de Herder WW, Dhatariya K, Dungan K, Hershman JM, Hofland J, Kalra S, Kaltsas G, Koch C, Kopp P, Korbonits M, Kovacs CS, Kuohung W, Laferrere B, Levy M, McGee EA, McLachlan R, Morley JE, New M, Purnell J, Sahay R, Singer F, Sperling MA, Stratakis CA, Trence DL, Wilson DP, eds. Endotext. South Dartmouth (MA)2000.
- Fukami M, Wada Y, Miyabayashi K, Nishino I, Hasegawa T, Nordenskjold A, Camerino G, Kretz C, Buj-Bello A, Laporte J, Yamada G, Morohashi K, Ogata T. CXorf6 is a causative gene for hypospadias. Nat Genet. 2006;38(12):1369-1371.
- Ogata T, Sano S, Nagata E, Kato F, Fukami M. MAMLD1 and 46,XY disorders of sex development. Semin Reprod Med. 2012;30(5):410-416.
- Beleza-Meireles A, Tohonen V, Soderhall C, Schwentner C, Radmayr C, Kockum I, Nordenskjold A. Activating transcription factor 3: a hormone responsive gene in the etiology of hypospadias. Eur J Endocrinol. 2008;158(5):729-739.
- Batool A, Karimi N, Wu XN, Chen SR, Liu YX. Testicular germ cell tumor: a comprehensive review. Cell Mol Life Sci. 2019;76(9):1713-1727.
- Moch H, Cubilla AL, Humphrey PA, Reuter VE, Ulbright TM. The 2016 WHO Classification of Tumours of the Urinary System and Male Genital Organs-Part A: Renal, Penile, and Testicular Tumours. Eur Urol. 2016;70(1):93-105.
- Berney DM, Looijenga LH, Idrees M, Oosterhuis JW, Rajpert-De Meyts E, Ulbright TM, Skakkebaek NE. Germ cell neoplasia in situ (GCNIS): evolution of the current nomenclature for testicular pre-invasive germ cell malignancy. Histopathology. 2016;69(1):7-10.
- Fink C, Baal N, Wilhelm J, Sarode P, Weigel R, Schumacher V, Nettersheim D, Schorle H, Schrock C, Bergmann M, Kliesch S, Kressin M, Savai R. On the origin of germ cell neoplasia in situ: Dedifferentiation of human adult Sertoli cells in cross talk with seminoma cells in vitro. Neoplasia.2021;23(7):731-742.
- Morin J, Peard L, Vanadurongvan T, Walker J, Donmez MI, Saltzman AF. Oncologic outcomes of pre-malignant and invasive germ cell tumors in patients with differences in sex development - A systematic review. J Pediatr Urol. 2020;16(5):576-582.
- Pierconti F, Martini M, Grande G, Larocca LM, Sacco E, Pugliese D, Gulino G, Bassi PF, Milardi D, Pontecorvi A. Germ Cell Neoplasia in situ (GCNIS) in Testis-Sparing Surgery (TSS) for Small Testicular Masses (STMs). Front Endocrinol (Lausanne). 2019;10:512.
- von der Maase H, Rorth M, Walbom-Jorgensen S, Sorensen BL, Christophersen IS, Hald T, Jacobsen GK, Berthelsen JG, Skakkebaek NE. Carcinoma in situ of contralateral testis in patients with testicular germ cell cancer: study of 27 cases in 500 patients. Br Med J (Clin Res Ed). 1986;293(6559):1398-1401.
- Jørgensen A, Lindhardt Johansen M, Juul A, Skakkebaek NE, Main KM, Rajpert-De Meyts E. Pathogenesis of germ cell neoplasia in testicular dysgenesis and disorders of sex development. Semin Cell Dev Biol. 2015;45:124-137.
- Baxter RM, Arboleda VA, Lee H, Barseghyan H, Adam MP, Fechner PY, Bargman R, Keegan C, Travers S, Schelley S, Hudgins L, Mathew RP, Stalker HJ, Zori R, Gordon OK, Ramos-Platt L, Pawlikowska-Haddal A, Eskin A, Nelson SF, Délot E, Vilain E. Exome sequencing for the diagnosis of 46,XY disorders of sex development. J Clin Endocrinol Metab. 2015;100(2):E333-344.
- Cools M, Pleskacova J, Stoop H, Hoebeke P, Van Laecke E, Drop SL, Lebl J, Oosterhuis JW, Looijenga LH, Wolffenbuttel KP, Group MC. Gonadal pathology and tumor risk in relation to clinical characteristics in patients with 45,X/46,XY mosaicism. J Clin Endocrinol Metab. 2011;96(7):E1171-1180.
- Spoor JA, Oosterhuis JW, Hersmus R, Biermann K, Wolffenbuttel KP, Cools M, Kazmi Z, Ahmed SF, Looijenga LHJ. Histological Assessment of Gonads in DSD: Relevance for Clinical Management. Sex Dev. 2018;12(1-3):106-122.
- Palma I, Garibay N, Pena-Yolanda R, Contreras A, Raya A, Dominguez C, Romero M, Aristi G, Queipo G. Utility of OCT3/4, TSPY and β-catenin as biological markers for gonadoblastoma formation and malignant germ cell tumor development in dysgenetic gonads. Dis Markers. 2013;34(6):419-424.
- Granados A, Alaniz VI, Mohnach L, Barseghyan H, Vilain E, Ostrer H, Quint EH, Chen M, Keegan CE. MAP3K1-related gonadal dysgenesis: Six new cases and review of the literature. Am J Med Genet C Semin Med Genet. 2017;175(2):253-259.
- Ferguson L, Agoulnik AI. Testicular cancer and cryptorchidism. Front Endocrinol (Lausanne). 2013;4:32.
- Leão R, Ahmad AE, Hamilton RJ. Testicular Cancer Biomarkers: A Role for Precision Medicine in Testicular Cancer. Clin Genitourin Cancer. 2019;17(1):e176-e183.
- Morin J, Peard L, Saltzman AF. Gonadal malignancy in patients with differences of sex development. Transl Androl Urol. 2020;9(5):2408-2415.
- Khan S, Mannel L, Koopman CL, Chimpiri R, Hansen KR, Craig LB. The use of MRI in the pre-surgical evaluation of patients with androgen insensitivity syndrome. J Pediatr Adolesc Gynecol.2014;27(1):e17-20.
- Rajpert-De Meyts E, Nielsen JE, Skakkebaek NE, Almstrup K. Diagnostic markers for germ cell neoplasms: from placental-like alkaline phosphatase to micro-RNAs. Folia Histochem Cytobiol.2015;53(3):177-188.
- Voorhoeve PM, le Sage C, Schrier M, Gillis AJ, Stoop H, Nagel R, Liu YP, van Duijse J, Drost J, Griekspoor A, Zlotorynski E, Yabuta N, De Vita G, Nojima H, Looijenga LH, Agami R. A genetic screen implicates miRNA-372 and miRNA-373 as oncogenes in testicular germ cell tumors. Cell.2006;124(6):1169-1181.
- Leao R, Albersen M, Looijenga LHJ, Tandstad T, Kollmannsberger C, Murray MJ, Culine S, Coleman N, Belge G, Hamilton RJ, Dieckmann KP. Circulating MicroRNAs, the Next-Generation Serum Biomarkers in Testicular Germ Cell Tumours: A Systematic Review. Eur Urol. 2021;80(4):456-466.
- Abaci A, Catli G, Berberoglu M. Gonadal malignancy risk and prophylactic gonadectomy in disorders of sexual development. J Pediatr Endocrinol Metab. 2015;28(9-10):1019-1027.
- Wünsch L, Holterhus PM, Wessel L, Hiort O. Patients with disorders of sex development (DSD) at risk of gonadal tumour development: management based on laparoscopic biopsy and molecular diagnosis. BJU Int. 2012;110(11 Pt C):E958-965.
- Barros BA, Oliveira LR, Surur CRC, Barros-Filho AA, Maciel-Guerra AT, Guerra-Junior G. Complete androgen insensitivity syndrome and risk of gonadal malignancy: systematic review. Ann Pediatr Endocrinol Metab. 2021;26(1):19-23.
- Patel V, Casey RK, Gomez-Lobo V. Timing of Gonadectomy in Patients with Complete Androgen Insensitivity Syndrome-Current Recommendations and Future Directions. J Pediatr Adolesc Gynecol.2016;29(4):320-325.
- Weidler EM, Linnaus ME, Baratz AB, Goncalves LF, Bailey S, Hernandez SJ, Gomez-Lobo V, van Leeuwen K. A Management Protocol for Gonad Preservation in Patients with Androgen Insensitivity Syndrome. J Pediatr Adolesc Gynecol. 2019;32(6):605-611.
- McNeill SA, O'Donnell M, Donat R, Lessells A, Hargreave TB. Estrogen secretion from a malignant sex cord stromal tumor in a patient with complete androgen insensitivity. Am J Obstet Gynecol.1997;177(6):1541-1542.
- Guercio G, Rey RA. Fertility issues in the management of patients with disorders of sex development. Endocr Dev. 2014;27:87-98.
- Van Batavia JP, Kolon TF. Fertility in disorders of sex development: A review. J Pediatr Urol.2016;12(6):418-425.
- Carson SA, Kallen AN. Diagnosis and Management of Infertility: A Review. JAMA. 2021;326(1):65-76.
- Foli KJ, VanGraafeiland B, Snethen JA, Greenberg CS. Caring for nontraditional families: Kinship, foster, and adoptive. J Spec Pediatr Nurs. 2022;27(3):e12388.
- King TF, Conway GS. Swyer syndrome. Curr Opin Endocrinol Diabetes Obes. 2014;21(6):504-510.
- Tordjman KM, Yaron M, Berkovitz A, Botchan A, Sultan C, Lumbroso S. Fertility after high-dose testosterone and intracytoplasmic sperm injection in a patient with androgen insensitivity syndrome with a previously unreported androgen receptor mutation. Andrologia. 2014;46(6):703-706.
- Matsubara K, Iwamoto H, Yoshida A, Ogata T. Semen analysis and successful paternity by intracytoplasmic sperm injection in a man with steroid 5α-reductase-2 deficiency. Fertil Steril.2010;94(7):2770.e2777-2710.
- Nordenskjöld A, Ivarsson SA. Molecular characterization of 5 alpha-reductase type 2 deficiency and fertility in a Swedish family. J Clin Endocrinol Metab. 1998;83(9):3236-3238.
- Bertelloni S, Baldinotti F, Baroncelli GI, Caligo MA, Peroni D. Paternity in 5α-Reductase-2 Deficiency: Report of Two Brothers with Spontaneous or Assisted Fertility and Literature Review. Sex Dev.2019;13(2):55-59.
- Guercio G, Costanzo M, Grinspon RP, Rey RA. Fertility Issues in Disorders of Sex Development. Endocrinol Metab Clin North Am. 2015;44(4):867-881.
- Finlayson C, Fritsch MK, Johnson EK, Rosoklija I, Gosiengfiao Y, Yerkes E, Madonna MB, Woodruff TK, Cheng E. Presence of Germ Cells in Disorders of Sex Development: Implications for Fertility Potential and Preservation. J Urol. 2017;197(3 Pt 2):937-943.
- Słowikowska-Hilczer J, Hirschberg AL, Claahsen-van der Grinten H, Reisch N, Bouvattier C, Thyen U, Cohen Kettenis P, Roehle R, Köhler B, Nordenström A, Group d-L. Fertility outcome and information on fertility issues in individuals with different forms of disorders of sex development: findings from the dsd-LIFE study. Fertil Steril. 2017;108(5):822-831.
- Oktay K, Harvey BE, Partridge AH, Quinn GP, Reinecke J, Taylor HS, Wallace WH, Wang ET, Loren AW. Fertility Preservation in Patients With Cancer: ASCO Clinical Practice Guideline Update. J Clin Oncol. 2018;36(19):1994-2001.
- Sadri-Ardekani H, Atala A. Testicular tissue cryopreservation and spermatogonial stem cell transplantation to restore fertility: from bench to bedside. Stem Cell Res Ther. 2014;5(3):68.
- Michel A, Mormont C, Legros JJ. A psycho-endocrinological overview of transsexualism. Eur J Endocrinol. 2001;145(4):365-376.
- Drescher J, Cohen-Kettenis P, Winter S. Minding the body: situating gender identity diagnoses in the ICD-11. Int Rev Psychiatry. 2012;24(6):568-577.
- Asscheman H, Giltay EJ, Megens JA, de Ronde WP, van Trotsenburg MA, Gooren LJ. A long-term follow-up study of mortality in transsexuals receiving treatment with cross-sex hormones. Eur J Endocrinol. 2011;164(4):635-642.
- Mustanski B, Liu RT. A longitudinal study of predictors of suicide attempts among lesbian, gay, bisexual, and transgender youth. Arch Sex Behav. 2013;42(3):437-448.
- Wylie K, Knudson G, Khan SI, Bonierbale M, Watanyusakul S, Baral S. Serving transgender people: clinical care considerations and service delivery models in transgender health. Lancet.2016;388(10042):401-411.
- Hembree WC, Cohen-Kettenis PT, Gooren L, Hannema SE, Meyer WJ, Murad MH, Rosenthal SM, Safer JD, Tangpricha V, T'Sjoen GG. Endocrine Treatment of Gender-Dysphoric/Gender-Incongruent Persons: An Endocrine Society Clinical Practice Guideline. J Clin Endocrinol Metab.2017;102(11):3869-3903.
- Hembree WC, Cohen-Kettenis P, Delemarre-van de Waal HA, Gooren LJ, Meyer WJ, 3rd, Spack NP, Tangpricha V, Montori VM, Endocrine S. Endocrine treatment of transsexual persons: an Endocrine Society clinical practice guideline. J Clin Endocrinol Metab. 2009;94(9):3132-3154.
- Costa EM, Mendonca BB. Clinical management of transsexual subjects. Arq Bras Endocrinol Metabol.2014;58(2):188-196.
- Toorians AW, Thomassen MC, Zweegman S, Magdeleyns EJ, Tans G, Gooren LJ, Rosing J. Venous thrombosis and changes of hemostatic variables during cross-sex hormone treatment in transsexual people. J Clin Endocrinol Metab. 2003;88(12):5723-5729.
- Jequier AM, Bullimore NJ, Bishop MJ. Cyproterone acetate and a small dose of oestrogen in the pre-operative management of male transsexuals. A report of three cases. Andrologia. 1989;21(5):456-461.
- Cunha FS, Domenice S, Camara VL, Sircili MH, Gooren LJ, Mendonca BB, Costa EM. Diagnosis of prolactinoma in two male-to-female transsexual subjects following high-dose cross-sex hormone therapy. Andrologia. 2015;47(6):680-684.
- Gooren LJ. Clinical practice. Care of transsexual persons. N Engl J Med. 2011;364(13):1251-1257.
- Wilson JD, Rivarola MA, Mendonca BB, Warne GL, Josso N, Drop SL, Grumbach MM. Advice on the management of ambiguous genitalia to a young endocrinologist from experienced clinicians. Semin Reprod Med. 2012;30(5):339-350.
- Achermann JC, Domenice S, Bachega TA, Nishi MY, Mendonca BB. Disorders of sex development: effect of molecular diagnostics. Nat Rev Endocrinol. 2015;11(8):478-488.
- Hiort O, Birnbaum W, Marshall L, Wünsch L, Werner R, Schröder T, Döhnert U, Holterhus PM. Management of disorders of sex development. Nat Rev Endocrinol. 2014;10(9):520-529.
- Bennecke E, Werner-Rosen K, Thyen U, Kleinemeier E, Lux A, Jürgensen M, Grüters A, Köhler B. Subjective need for psychological support (PsySupp) in parents of children and adolescents with disorders of sex development (dsd). Eur J Pediatr. 2015;174(10):1287-1297.
- Markosyan R, Ahmed SF. Sex Assignment in Conditions Affecting Sex Development. J Clin Res Pediatr Endocrinol. 2017;9(Suppl 2):106-112.
- Sandberg DE, Callens N, Wisniewski AB. Disorders of Sex Development (DSD): Networking and Standardization Considerations. Horm Metab Res. 2015;47(5):387-393.
- Streuli JC, Vayena E, Cavicchia-Balmer Y, Huber J. Shaping parents: impact of contrasting professional counseling on parents' decision making for children with disorders of sex development. J Sex Med.2013;10(8):1953-1960.
- Ediati A, Maharani N, Utari A. Sociocultural aspects of disorders of sex development. Birth Defects Res C Embryo Today. 2016;108(4):380-383.
- Moshiri M, Chapman T, Fechner PY, Dubinsky TJ, Shnorhavorian M, Osman S, Bhargava P, Katz DS. Evaluation and management of disorders of sex development: multidisciplinary approach to a complex diagnosis. Radiographics. 2012;32(6):1599-1618.
- Massanyi EZ, Dicarlo HN, Migeon CJ, Gearhart JP. Review and management of 46,XY disorders of sex development. J Pediatr Urol. 2013;9(3):368-379.
- Lee PA, Houk CP, Ahmed SF, Hughes IA. Consensus statement on management of intersex disorders. International Consensus Conference on Intersex. Pediatrics. 2006;118(2):e488-500.
- Hewitt J, Zacharin M. Hormone replacement in disorders of sex development: Current thinking. Best Pract Res Clin Endocrinol Metab. 2015;29(3):437-447.
- Birnbaum W, Bertelloni S. Sex hormone replacement in disorders of sex development. Endocr Dev.2014;27:149-159.
- Crandall CJ, Hovey KM, Andrews C, Cauley JA, Stefanick M, Shufelt C, Prentice RL, Kaunitz AM, Eaton C, Wactawski-Wende J, Manson JE. Comparison of clinical outcomes among users of oral and transdermal estrogen therapy in the Women's Health Initiative Observational Study. Menopause.2017;24(10):1145-1153.
- Adami S, Rossini M, Zamberlan N, Bertoldo F, Dorizzi R, Lo Cascio V. Long-term effects of transdermal and oral estrogens on serum lipids and lipoproteins in postmenopausal women. Maturitas.1993;17(3):191-196.
- Ankarberg-Lindgren C, Kriström B, Norjavaara E. Physiological estrogen replacement therapy for puberty induction in girls: a clinical observational study. Horm Res Paediatr. 2014;81(4):239-244.
- Cools M, Nordenström A, Robeva R, Hall J, Westerveld P, Flück C, Köhler B, Berra M, Springer A, Schweizer K, Pasterski V, 1 CABwg. Caring for individuals with a difference of sex development (DSD): a Consensus Statement. Nat Rev Endocrinol. 2018;14(7):415-429.
- Schonbucher V, Schweizer K, Richter-Appelt H. Sexual quality of life of individuals with disorders of sex development and a 46,XY karyotype: a review of international research. J Sex Marital Ther.2010;36(3):193-215.
- Minto CL, Liao KL, Conway GS, Creighton SM. Sexual function in women with complete androgen insensitivity syndrome. Fertil Steril. 2003;80(1):157-164.
- Birnbaum W, Marshall L, Werner R, Kulle A, Holterhus PM, Rall K, Köhler B, Richter-Unruh A, Hartmann MF, Wudy SA, Auer MK, Lux A, Kropf S, Hiort O. Oestrogen versus androgen in hormone-replacement therapy for complete androgen insensitivity syndrome: a multicentre, randomised, double-dummy, double-blind crossover trial. Lancet Diabetes Endocrinol. 2018;6(10):771-780.
- Batista RL, Mendonca BB. Testosterone replacement in androgen insensitivity: is there an advantage? Ann Transl Med. 2018;6(Suppl 1):S85.
- Khen-Dunlop N, Lortat-Jacob S, Thibaud E, Clement-Ziza M, Lyonnet S, Nihoul-Fekete C. Rokitansky syndrome: clinical experience and results of sigmoid vaginoplasty in 23 young girls. J Urol.2007;177(3):1107-1111.
- Werner R, Grötsch H, Hiort O. 46,XY disorders of sex development--the undermasculinised male with disorders of androgen action. Best Pract Res Clin Endocrinol Metab. 2010;24(2):263-277.
- McGriff NJ, Csako G, Kabbani M, Diep L, Chrousos GP, Pucino F. Treatment options for a patient experiencing pruritic rash associated with transdermal testosterone: a review of the literature. Pharmacotherapy. 2001;21(11):1425-1435.
- El-Maouche D, Arlt W, Merke DP. Congenital adrenal hyperplasia. Lancet. 2017;390(10108):2194-2210.
- Fleming L, Van Riper M, Knafl K. Management of Childhood Congenital Adrenal Hyperplasia-An Integrative Review of the Literature. J Pediatr Health Care. 2017;31(5):560-577.
- Mouriquand PD, Gorduza DB, Gay CL, Meyer-Bahlburg HF, Baker L, Baskin LS, Bouvattier C, Braga LH, Caldamone AC, Duranteau L, El Ghoneimi A, Hensle TW, Hoebeke P, Kaefer M, Kalfa N, Kolon TF, Manzoni G, Mure PY, Nordenskjöld A, Pippi Salle JL, Poppas DP, Ransley PG, Rink RC, Rodrigo R, Sann L, Schober J, Sibai H, Wisniewski A, Wolffenbuttel KP, Lee P. Surgery in disorders of sex development (DSD) with a gender issue: If (why), when, and how? J Pediatr Urol. 2016;12(3):139-149.
- Creighton S, Chernausek SD, Romao R, Ransley P, Salle JP. Timing and nature of reconstructive surgery for disorders of sex development - introduction. J Pediatr Urol. 2012;8(6):602-610.
- Bennecke E, Bernstein S, Lee P, van de Grift TC, Nordenskjöld A, Rapp M, Simmonds M, Streuli JC, Thyen U, Wiesemann C, Group d-L. Early Genital Surgery in Disorders/Differences of Sex Development: Patients' Perspectives. Arch Sex Behav. 2021;50(3):913-923.
- Sircili MH, de Mendonca BB, Denes FT, Madureira G, Bachega TA, e Silva FA. Anatomical and functional outcomes of feminizing genitoplasty for ambiguous genitalia in patients with virilizing congenital adrenal hyperplasia. Clinics. 2006;61(3):209-214.
- Dénes FT, Cocuzza MA, Schneider-Monteiro ED, Silva FA, Costa EM, Mendonca BB, Arap S. The laparoscopic management of intersex patients: the preferred approach. BJU Int. 2005;95(6):863-867.
- Bernabé KJ, Nokoff NJ, Galan D, Felsen D, Aston CE, Austin P, Baskin L, Chan YM, Cheng EY, Diamond DA, Ellens R, Fried A, Greenfield S, Kolon T, Kropp B, Lakshmanan Y, Meyer S, Meyer T, Delozier AM, Mullins LL, Palmer B, Paradis A, Reddy P, Reyes KJS, Schulte M, Swartz JM, Yerkes E, Wolfe-Christensen C, Wisniewski AB, Poppas DP. Preliminary report: Surgical outcomes following genitoplasty in children with moderate to severe genital atypia. J Pediatr Urol. 2018;14(2):157.e151-157.e158.
- Jesus LE. Feminizing genitoplasties: Where are we now? J Pediatr Urol. 2018;14(5):407-415.
- FORTUNOFF S, LATTIMER JK, EDSON M. VAGINOPLASTY TECHNIQUE FOR FEMALE PSEUDOHERMAPHRODITES. Surg Gynecol Obstet. 1964;118:545-548.
- Sircili MH, Bachega TS, Madureira G, Gomes L, Mendonca BB, Dénes FT. Surgical Treatment after Failed Primary Correction of Urogenital Sinus in Female Patients with Virilizing Congenital Adrenal Hyperplasia: Are Good Results Possible? Front Pediatr. 2016;4:118.
- Baskin LS, Erol A, Li YW, Liu WH, Kurzrock E, Cunha GR. Anatomical studies of the human clitoris. J Urol. 1999;162(3 Pt 2):1015-1020.
- Kogan SJ, Smey P, Levitt SB. Subtunical total reduction clitoroplasty: a safe modification of existing techniques. J Urol. 1983;130(4):746-748.
- Rink RC, Cain MP. Urogenital mobilization for urogenital sinus repair. BJU Int. 2008;102(9):1182-1197.
- Sircili MH, de Mendonca BB, Denes FT, Madureira G, Bachega TA, e Silva FA. Anatomical and functional outcomes of feminizing genitoplasty for ambiguous genitalia in patients with virilizing congenital adrenal hyperplasia. Clinics (Sao Paulo). 2006;61(3):209-214.
- Kolon TF, Herndon CD, Baker LA, Baskin LS, Baxter CG, Cheng EY, Diaz M, Lee PA, Seashore CJ, Tasian GE, Barthold JS, Assocation AU. Evaluation and treatment of cryptorchidism: AUA guideline. J Urol. 2014;192(2):337-345.
- Sircili MH, Denes FT, Costa EM, Machado MG, Inacio M, Silva RB, Srougi M, Mendonca BB, Domenice S. Long-term followup of a large cohort of patients with ovotesticular disorder of sex development. J Urol. 2014;191(5 Suppl):1532-1536.
- Pippi Salle JL, Sayed S, Salle A, Bagli D, Farhat W, Koyle M, Lorenzo AJ. Proximal hypospadias: A persistent challenge. Single institution outcome analysis of three surgical techniques over a 10-year period. J Pediatr Urol. 2016;12(1):28.e21-27.
- Snodgrass WT, Granberg C, Bush NC. Urethral strictures following urethral plate and proximal urethral elevation during proximal TIP hypospadias repair. J Pediatr Urol. 2013;9(6 Pt B):990-994.
- Romao RLP, Pippi Salle JL. Update on the surgical approach for reconstruction of the male genitalia. Semin Perinatol. 2017;41(4):218-226.
- Steven L, Cherian A, Yankovic F, Mathur A, Kulkarni M, Cuckow P. Current practice in paediatric hypospadias surgery; a specialist survey. J Pediatr Urol. 2013;9(6 Pt B):1126-1130.
- Hafez AT, Helmy T. Tubularized incised plate repair for penoscrotal hypospadias: role of surgeon's experience. Urology. 2012;79(2):425-427.
- Cools M, Looijenga LH, Wolffenbuttel KP, T'Sjoen G. Managing the risk of germ cell tumourigenesis in disorders of sex development patients. Endocr Dev. 2014;27:185-196.
- Lee P, Schober J, Nordenström A, Hoebeke P, Houk C, Looijenga L, Manzoni G, Reiner W, Woodhouse C. Review of recent outcome data of disorders of sex development (DSD): emphasis on surgical and sexual outcomes. J Pediatr Urol. 2012;8(6):611-615.
- Ark JT, Moses KA. Operative considerations for late-presenting persistent Müllerian duct syndrome. Urol Ann. 2016;8(3):363-365.
- Andersson M, Sjöström S, Wängqvist M, Örtqvist L, Nordenskjöld A, Holmdahl G. Psychosocial and Sexual Outcomes in Adolescents following Surgery for Proximal Hypospadias in Childhood. J Urol.2018;200(6):1362-1370.
- Rynja SP, de Jong TP, Bosch JL, de Kort LM. Functional, cosmetic and psychosexual results in adult men who underwent hypospadias correction in childhood. J Pediatr Urol. 2011;7(5):504-515.
- van der Zwan YG, Callens N, van Kuppenveld J, Kwak K, Drop SL, Kortmann B, Dessens AB, Wolffenbuttel KP, DSD DSGo. Long-term outcomes in males with disorders of sex development. J Urol.2013;190(3):1038-1042.
- Köhler B, Kleinemeier E, Lux A, Hiort O, Grüters A, Thyen U, Group DNW. Satisfaction with genital surgery and sexual life of adults with XY disorders of sex development: results from the German clinical evaluation study. J Clin Endocrinol Metab. 2012;97(2):577-588.
- Meyer-Bahlburg HF, Migeon CJ, Berkovitz GD, Gearhart JP, Dolezal C, Wisniewski AB. Attitudes of adult 46, XY intersex persons to clinical management policies. J Urol. 2004;171(4):1615-1619; discussion 1619.
- Mureau MA, Slijper FM, van der Meulen JC, Verhulst FC, Slob AK. Psychosexual adjustment of men who underwent hypospadias repair: a norm-related study. J Urol. 1995;154(4):1351-1355.
- Bubanj TB, Perovic SV, Milicevic RM, Jovcic SB, Marjanovic ZO, Djordjevic MM. Sexual behavior and sexual function of adults after hypospadias surgery: a comparative study. J Urol. 2004;171(5):1876-1879.